December 31, 2007
| Inventory of 2007 MR events and books
Magnetic resonance being a high-speed roller-coster, it is beyond one man's capabilities to take its snapshot. All I can do to measure its pulse is to list the number of MR events (conferences, symposia, meetings, ...) which took place during 2007 and the number of books which were published. Included are also the respective partial counts for 2008 based on confirmed data available at this moment.
Events: | 2007 | Notes: | slated for 2008 |
NMR | 61 | Includes all but medical imaging | 19 |
ESR | 12 | EPR is on an upswing | 4 |
MRI | 28 | Some of these meetings are huge | 5 |
Related | 19 | This category is very incomplete | 16 |
Total | 120 | About one every 3 days! | |
| | | |
Books: | 2007 | Notes: | slated for 2008 |
NMR | 14 | Within average | 5 |
ESR | 3 | Weak, but 2006 was strong | 1 |
MRI | 31 | Medical MRI texts are shooting up | 18 |
I thank all those who have let me know about forthcoming events and newly published books as well as those who will do so in the future. Announcements of events and books on this site are free of any charge and represent both a promotional vehicle and a service to the MR community.
|
December 30, 2007
SEQUELS:
13 Jun 2008
22 Mar 2009
ERRATA
W.Proctor & F.C.Yu
Physical Review
77, p.717, 1950.
14N at 3.3 MHz
in NH4NO3
Chemical shift:
about 485 ppm.
W.C.Dickinson
Physical Review
77, p.736, 1950.
19F at 28 MHz.
SbF3 + BeF2
Chemical shift:
about 117 ppm.
Magnetic field
inhomogeneity of
20-100 ppm made
the small 1H shifts
unobservable
(Dickinson tried)
while sensitivity
was not an issue!
All samples were
heavily doped
solutions.
W.G.Proctor,
F.C.Yu
Physical Review
81, p.20, 1951.
121Sb at 6.7 MHz
in NaSbF6.
Sb-F J-coupling:
about 1930 Hz.
They thought that
the pattern was due
to dipole-dipole
interactions!
W.C.Dickinson
and W.G.Proctor,
a continent apart,
were informed
about each other's
progress by
Francis Bitter,
the magnet man.
| Chemical Shifts and Shyness
Since some time I am kind of obsessed by an apparent paradox. In many cases we know a lot of biographical details about minor players in the fascinating story of nuclear magnetic resonance. Quite correctly, we also honor and invite to meetings nearly everybody who has achieved anything worth honoring. Yet when it comes to the three discoverers of chemical shifts, W.G.Proctor, F.C.Yu and W.C.Dickinson, the available bigraphical knowledge is very scarce and I wonder how many of us have ever met any of them at a meeting (not me, and I was sticking around for over 40 years).
During this year emerged a few pieces of info about Warren George Proctor who, at the time of the discovery, worked together with Fu Chun Yu at Felix Bloch's laboratory at Stanford University. The discovery itself is vividly captured in the now public Warren's memo.
Last April, as a reaction to the memo and to my comments, I have received an e-mail from Judith Shoolery, the wife of James N.Shoolery and the author of Edward Teller Memoirs. She wrote: "Warren moved to Europe about 1974, teaching first at University of Grenoble. I believe that was his home when his article on astrophysics was published as the lead article in Scientific American. I am not certain of the other posts he had on the continent, but he died in 2006 in Goussarges, France.". In a subsequent e-mail she added: "Warren and his wife were our good friends". Combining Judith's hints with affiliations reported in Warren's papers, I was able to stitch together the following picture:
After the startup at Felix Bloch's laboratory [1-8] with F.C.Yu and M.Packard, Warren got in contact [9 (1951)] with University of Basel (Switzerland). Before concluding a contract with them [11 (1956)], however, he went through University of Washington [10-13 (1955-6)], working on interactions between ultrasound and spin systems. Somewhere at that point [13-14 (1956)] he was hired by Varian Associates in Palo Alto. That must be when he made friends with Jim Shoolery, the man who fashioned NMR into a tool for chemists. He than moved overseas, working with Anatol Abragam in Saclay, France, on the concept of spin temperature [15-16 (1957-8)], a topic which captured his fancy [20]. While at Saclay, he must have been still on Varian payroll (or a leave) because thereafter [17-18] we find him at the Varian facility in Zug, Switzerland (last paper with that affiliation is of 1967). Later, he must have returned to Palo Alto [19 (1972)]. Judith's comment that he "moved to Europe in 1974" is probably not precise since Warren himself [21] indicates 1975 as the year he left Varian and specifies that he was the Managing Director of the Zug facility (the company is not very good at digging this out of their records). I was so far unable to substantiate his association with Uni Grenoble. Rather, he writes [21] that he worked at University of Helsinky and then the Technical University of Denmark, and retired in 1986. The 'astrophysics' paper Judith mentions must have been ref [20 (1978)] since that appears to be the only article he published in Scientific American (alas, it carries no affiliation).
I have managed to track the tiny French village Judith mentions; its spelling is Goussargues, and it is a fraction of Goudargues, located in Département du Gard, close to Verfuil. The Mayor of Goudargues, E.Taulelle, confirmed to me in a letter dated July 2, 2007, that "Monsieur Proctor Warren died in 2005 and is buried in Goussargues". Evidently, Judith made a slight error in the date, but that might happen if the death occurred at the end of the year.
So, since two years, one of the fathers of chemical shifts rests in peace in Provence.
What strikes me most in this story is the fact that for nearly one third of his life, Warren just went incognito. There were ever more numerous NMR conferences being held all around and nobody knew he lived close by. With what chemical shifts mean today, if he just said "hello, I am here", he would have been invited as a honored guest to all of them.
The destinies of Fu Chun Yu remain, for me, a complete mystery, except for what Warren wrote about their friendship in ref.[21]. W.C.Dickinson, after his promising start in NMR [1-6 (1949-51)], moved to the Los Alamos project [7-8]. After publishing one more paper with Lawrence Radiation Laboratory [9 (1962)], he became totally silent and I can't find any hint of what became of him. So, like Proctor, both Yu and Dickinson dropped out of public view and never reclaimed their part of the glory.
I can't help but ask: is this something chemical shifts do to people ?
All papers by W.G.Proctor (and F.C.Yu) I could find (in chronological order):
Notes following three stars indicate a change in affiliation.
- Proctor W.G.,
The Magnetic Moment of Tl203,
Phys.Rev. 75, 522 (1949).
*** Department of Physics, Stanford University, California
- Proctor W.G.,
The Magnetic Moments of Sn117, Sn119, and Pb207,
Phys.Rev. 76, 684 (1949).
- Proctor W.G., Yu F.C.,
On the Magnetic Moments of Sn115, Cd111, Cd113, Pt195, and Hg199,
Phys.Rev. 76, 1728 (1949).
DOI: 10.1103/PhysRev.76.1728.3.
- Proctor W.G., Yu F.C.,
On the Magnetic Moments of Mn55, Co59, Cl37, N15, and N14,
Phys.Rev. 77, 716 (1950).
DOI: 10.1103/PhysRev.77.716.
- Proctor W.G., Yu F.C.,
The Dependence of a Nuclear Magnetic Resonance Frequency upon Chemical Compounds,
Phys.Rev. 77, 717 (1950).
DOI: 10.1103/PhysRev.77.717.
Discovery of chemical shifts (14N and 15N).
Read Proctor's personal account.
- Proctor W.G., Yu F.C.,
On the Magnetic Moments of Xe129, Bi209, Sc45, Sb121, and Sb123,
Phys.Rev. 78, 471 (1950).
DOI: 10.1103/PhysRev.78.471.
- Proctor W.G.,
On the Magnetic Moments of Tl203, Tl205, Sn115, Sn117, Sn119, Cd111, Cd113, and Pb207,
Phys.Rev. 79, 35-44 (1950).
Technical description of the "Proctor" NMR instrument.
- Proctor W.G., Yu F.C.,
On the Nuclear Magnetic Moments of Several Stable Isotopes,
Phys.Rev. 81, 20-30 (1951).
DOI: 10.1103/PhysRev.81.20.
First observation of scalar coupling (wrong explanation).
Read Proctor's personal account.
- Baldinger E., Huber P., Proctor W.G.,
Scattering of Fast Neutrons from O16,
Phys.Rev. 84, 1058 (1951).
*** Physikalische Anstalt der Universität Basel, Basel, Switzerland.
*** Proctor still at Stanford University, California
- Proctor W.G., Tanttila W.H.,
Saturation of Nuclear Electric Quadrupole Energy Levels by Ultrasonic Excitation,
Phys.Rev. 98, 1854 (1955).
*** Department of Physics, University of Washington, Seattle, Washington.
- Proctor W.G., Tanttila W.H.,
Influence of Ultrasonic Energy on the Relaxation of Chlorine Nuclei in Sodium Chlorate,
Phys.Rev. 101, 1757 (1956).
*** Department of Physics, University of Washington, Seattle, Washington.
*** Proctor now at Universität Basel, Basel, Switzerland.
- Proctor W.G., Robinson W.A.,
Ultrasonic Saturation of Nuclear Magnetic Energy Levels,
Phys.Rev. 102, 1183 (1956).
- Kiddle R.F., Proctor W.G.,
Nuclear Quadrupole Interactions in Two Tutton's Salts,
Phys.Rev. 104, 932 (1956).
*** Department of Physics, University of Washington, Seattle, Washington.
*** Proctor now at Varian Associates, Palo Alto, California
- Proctor W.G., Robinson W.A.,
Ultrasonic Excitation of Nuclear Magnetic Energy Levels of Na23 in NaCl,
Phys.Rev. 104, 1344 (1956).
- Abragam A., Proctor W.G.,
Experiments on Spin Temperature,
Phys.Rev. 106, 160-161 (1957).
The spin temperature concept remained controversial and not very fertile.
*** Centre d'Études Nucléaires de Saclay, Gif-sur-Yvette (Seine-et-Oise), France.
- Abragam A., Proctor W.G.,
Spin Temperature,
Phys.Rev. 109, 1441-1458 (1958).
- Lee K., Sher A., Andersson L.O., Proctor W.G.,
Temperature Variation of La139 Nuclear Quadrupole Resonance in LaF3,
Phys.Rev. 150, 168 (1966).
*** Varian AG, Zürich, Switzerland (the town was actually Zug)
- Mahon H., Brun E., Lukkala M., Proctor W.G.,
Excitation of Fractional Harmonic Phonons in Solids,
Phys.Rev.Lett. 19, 430 (1967).
- Wood C., Shaffer J.C., Proctor W.G.,
Electron Energy States in Sb2Se3,
Phys.Rev.Lett. 29, 485 (1972).
*** Instrument Division, Varian Associates, Palo Alto, California.
- Proctor W.G.,
Negative Absolute Temperatures,
Scientific American 239(2), 90-99 (August 1978). No affiliation indicated.
Abstract: No substance can be cooled below absolute zero, but some physical systems can have a negative temperature on an absolute scale. Such temperatures are not colder than zero but are hotter than infinity.
- Proctor W.G.,
Proctor Warren G.: When You and I Were Young, Magnet,
in Encyclopedia of Magnetic Resonance,
Historical Perspectives,
J.Wiley 2002. ISBN 0-470-84784-8. On-line version updated in 2007.
All papers by W.C.Dickinson I could find:
- Dickinson W.C., Wimett T.F.,
The Magnetic Moment of Be9,
Phys.Rev. 75, 1769 (1949).
*** Research Laboratory of Electronics, MIT, Cambridge
- Dickinson W.C.,
Magnetic Moment of La139,
Phys.Rev. 76, 1414 (1949).
- Dickinson W.C.,
Dependence of the F19 Nuclear Resonance Position on Chemical Compound,
Phys.Rev. 77, 736-737 (1950).
Parallel discovery of chemical shifts (19F).
- Bloembergen N., Dickinson W.C.,
On the Shift of the Nuclear Magnetic Resonance in Paramagnetic Solutions,
Phys.Rev. 79, 179-180 (1950).
- Dickinson W.C.,
Hartree Computation of the Internal Diamagnetic Field for Atoms,
Phys.Rev. 80, 563 (1950).
One of the first explanations of chemical shifts.
- Dickinson W.C.,
The Time Average Magnetic Field at the Nucleus in Nuclear Magnetic Resonance Experiments,
Phys.Rev. 81, 717-731 (1951).
Early estimates of chemical shifts.
- Brolley J.E., Dickinson W.C.,
Angular Distribution of Fragments from Neutron-Induced Fission,
Phys.Rev. 94, 640 (1954).
*** Los Alamos Scientific Laboratory, University of California, Los Alamos.
- Brolley J.E., Dickinson W.C., Henkel R.L.,
Angular Dependence of the Neutron-Induced Fission Process. II,
Phys.Rev. 99, 159 (1955).
- Dickinson W.C., Passell L., Halpern O.,
Studies of the Optics of Neutrons. I. Measurement of the Neutron-Proton Coherent Scattering Amplitude by Mirror Reflection, Phys.Rev. 126, 632 (1962).
*** Lawrence Radiation Laboratory, University of California, Livermore.
ERRATA CORRIGE:
On November 17, 2008, I have received from Judith Shoolery the following e-mail which adds additional details about Warren Proctor's final resting place:
Dear Stan, I enjoyed hearing from you again.
However, I unintentionally misled you about where Warren is buried. His ashes lie in front of his home for the last twenty years or so in a little Danish village near Copenhagen overlooking the sea, with a stele to mark his grave. Because his step-family still lives in the house, I am not comfortable sharing the exact address without their permission. He died in the village in France, but his step-family brought his ashes back to the family home.
With warm good wishes, Judy Shoolery.
Those wishing to follow the trail further might find the name of the Danish village in the last Warren's writings but that, I think, would hardly add anything to his great memory.
|
December 21, 2007
| The NMR Spectroscopy Army rents combat stations!
Naohiro Kobayashi of the RIKEN facility (see the last entry), while letting me know about their new web page dedicated to the free (for academics) KUJIRA BioNMR software, added the following comment which, I believe, is of a more general interest:
... I have also enjoyed your news blog, and I believe you have been impressed how magnificent our facility is. But actually the project, Protein 3000 is finished and our NMR machines are now open to all structure biologists in Japanese academic and commercial societies. ...
Since I am currently trying to sort out the 'worldwide' prices for rented NMR machine time, Naohiro's last words aroused my interest. There is a page on the RIKEN site which is in Japanese, but terminates with an ASCI contact e-mail address. And it carries the prices which a Japanese friend of mine deciphered for me: they range from 700$ (at 600 MHz) to 1300$ (900 MHz) per week.
This is not just much less than what we pay in Europe (shame on the so-called public-access policies of the EU!). More important is the fact that, for once, this really is affordable (even for me). If, for example, I had a project which required the use of an 800 MHz machine for one whole month, I could make it for 6000 euros: 4000 the machine time + some help, 1000 for the return ticket to Japan (low cost), and 1000 for local travel and living expenses. I do not know (yet) whether the above prices apply only to Japanese customers but, if I were interested, I would get in contact with RIKEN very fast - before the waiting lines become too long.
The NMR access market is becoming global. Get smart, gents!
|
December 19, 2007
Total:
29.2 GHz !
| An Invincible NMR Spectroscopy Army
Did you think that the handful of European Large Scale NMR Facilities were a big thing? Or the New York Structural Biology Center with its eight instruments in a single room? If so, have a look at the NMR Complex of the Genomics Sciences Center of RIKEN Yokohama Research Institute (Japan).
I admit that before I had seen this page, I could have never imagined 42 instruments in a single facility, all of them but two 600 MHz or higher (three 900's)! I do not think that anything even distantly comparable exists anywhere in the USA or in Europe. Clearly, the Japanese do things in a big way nowadays.
It is interesting that this national protein and genomics research project bets almost all on NMR which has apparently absorbed most of the investment. It has been proposed in 1995 and became fully operative about five years ago. The organization is also interesting. The director of the project is Shigeyuki Yokoyama, a biochemist and biophysicist, and they have four NMR teams: NMR Spectroscopy Research Team (Toshio Yamazaki, previously with Jeol), NMR Technology Research Team (Hideaki Maeda, previously with Toshiba), NMR Pipeline Application Research Team (Fumiaki Hayashi), and NMR Pipeline Methodology Research Team (Takanori Kigawa).
Somehow, it seems, magnetic resonance appeals to the Japanese psyche. It already turned out that Japan has the highest pro-capite density of MRI imagers in the world and now I would not be surprised to find out that the same is true also about NMR spectrometers.
|
December 17, 2007
| More on coils versus antennas - and NQR
Vladimir Korostelev writes:
Dear Stan,
... The rf antennas used for NQR remote detection of landmines are ferrite antennas and, technically, have nothing to do with coils. Coils are used for detection of explosives in a closed volume, in a bag, for example. ...
Ok, Vladimir, I take your word for it. However, this is not quite pertinent to what I am discussing, which is that some aspects of MR transmitter/receiver coils (the lack of manifest radiation and the way they are constructed) make them different from antennas. The point is not at all whether antennas were, or will be, used somewhere in MR (I hope they will) but rather whether
the coils of standard NMR/MRI can be considered antennas and, if not, how comes that quantum effects are still fully functional.
In addition, NQR in Earth magnetic field is not a magnetic resonance at all since there the nuclides precess around intra-molecular electric field gradients, not about the external magnetic field which is just a minor perturbation. Conceptually, that kind of spectroscopy resembles more the microwave spectroscopy of rotating dipolaral molecules than NMR.
After all, as its name indicates, pure Nuclear Quadrupole Resonance is a nuclear resonance, not a magnetic one, notwithstanding the fact that, applying an external magnetic field of increasing strength one can achieve a continuous transition from NQR (with magnetic perturbations) to NMR (with quadrupole perturbations). Incidentally, in all such contexts, as far as I know, transmit/receive coils are again ubiquitous.
By the way, I did contact David Hoult and received his prompt answer. He pointed out to me that one important reference was missing in my article on the Reciprocity Theorem. Here it is: Hoult D.I., Ginsberg N.S., The Quantum Origins of the Free Induction Decay Signal and Spin Noise, J.Magn.Reson. 148, 182-199 (2001). Have a look at it, it is a great reading.
|
December 15, 2007
MR spectroscopy
with true antennas
was never done !?
Lumped version:
MR coil(s)/cavity.
Where is radiation!?
Quantum physics
still works:
photons are OK
and in good health
Remote sensing:
Sample must be still
close to the coil!
Is this an antenna?
Sure, and babies
are born under
cauliflowers!
| Coils versus Antennas
I have received the following e-mail in response to the entry on Sakellariou MACS rotor and the article on Antenna Reciprocity Theorem in Magnetic Resonance:
Dear Dr Stan Sykora,
I have noticed in your blog that
"The situation has changed after David I.Hoult has shown that the nuclear induction experiment has actually little to do with classical induction and a lot with RF antennas and the way magnetic dipoles interact with the magnetic component of their electromagnetic fields".
Actually, there is a subfield of NMR and NQR which has relied on RF antennas long before D.I.Hoult's work. This is used for landmine detection using either nitrogen NMR or NQR of explosive placed inside of landmines.
With best wishes, Vladimir Korostelev
Dear Vladimir, this is a coincidence since I a preparing a long-planned entry on Magnetic Resonance and Warfare where remote detection of explosives will play a major role. I was in fact already aware of your name, as well as of your and V.S.Grechishkin's patent.
Let me first point out that your comment does not contradict Hoult's work, nor his MR reciprocity theorem (in fact, you do not claim so). In my opinion, however, your comment is slightly misleading, though not really erroneous. There are some aspects of the coil-versus-antenna controversy which indicate that the MR transmit/receive devices are much closer to induction coils than to antennas, despite the term antenna is in common use in some application areas, such as the one you have mentioned and, even more so, clinical MRI. I will now try and wrap up my own opinions about this controversial topic.
In the common idiom, an antenna is any remote sensing device, such as the antennae of some insects or marine creatures. In such a picturesque sense, one can call antenna a lot of things - one just should not mention it in the presence of those who build real RF antennas.
Technically, an antenna is a device which converts RF power stored in a circuit into a freely propagating electromagnetic (EM) radiation, or vice versa. Once produced, an EM radiation propagates through free space on its own until absorbed/dispersed by an object. While doing so, its intensity drops with the square of distance and the decay can be made arbitrarily slow by shaping it as a narrow beam. A crucial role in the process plays the characteristic impedance of vacuum Z0 = 376.73 Ω which matches also the ratio |E|/|H| between the orthogonal electric and magnetic intensity vectors of any EM wave. To be efficient, an antenna must (a) transform its input impedance (defined, for example, by a 50 Ω coaxial cable) into its output impedance represented by the vacuum, which is a 377 Ω EM-radiation conductor, and (b) generate synchronously oscillating, orthogonal electric and magnetic fields with the proper ratio between their intensities. These are the basics of the fine art of antenna design.
Now, none of this art is used in MR coils! We only care about the magnetic field and not about the electric field component. There is a little bit of the latter, associated with dB/dt, but it is far too small for an efficient antenna. Consequently, our coils generate very little radiation - a reason why 2 kW decouplers do not violate EMI regulations and why you can not detect NMR signals by placing an antenna on the ceiling above a supercon spectrometer (I tried!). Actually, we do not want to radiate! All we want are local magnetic fields. From far away, our coils approximate magnetic dipoles, so that the generated field (and, by the reciprocity theorem, the device sensitivity) drops with the cube of distance. Though the induction principle makes contact-less sensing possible, the 'sample' still needs to be pretty close to the coil for the signal to be detectable (as you sure know, the useful distance is comparable with the coil dimensions). This applies to all NMR coils, inluding those for MRI, NQR remote-sensing, and the >100 m wide monsters used for geo-MRI. They, just like beach metal detectors, are no true antennas!
What D.I.Hoult has done, in my opinion, are two things:
(1) He pointed out a basic difference between MR and other EM spectroscopies.
In general, we have the scheme source=>photons=>sample=>photons=>detector. Since the photons have a life of their own, spectroscopic phenomena can be simplified by considering only the sub-scheme photons=>sample=>photons (as an extreme case, think about a star as a source and an intervening galactic dust cloud as a sample). In magnetic resonance, however, the scheme is tx_coil=>sample=>rx_coil, with the additional complication that in many arrangements the two coils actually coincide. There are no radiated photons and the source and detector are lumped together, forming either a single coil or a crossed-coils assembly, or a resonant cavity. In this situation, excitation and detection are often described by means of conventional induction laws which, however, are inadequate to describe the quantum character of the phenomenon (they are inadequate also for other reasons which exceed the scope of this entry). The existence of a spectral line 0.05 Hz wide at 500 MHz (a de-facto quality factor of 1e10) cannot be described by anything classical, yet the only electromagnetic quanta (photons) we can think about in this situation are virtual. Hoult did not solve this impasse, he just pointed it out and speculated about how it might be tackled.
(2) He modified the classical antenna reciprocity theorem to make it applicable and useful in the MR context. Actually, for the same reasons discussed above, the result should no longer be called antenna theorem at all, just MR reciprocity theorem or Hoult's theorem. In this sense, I have erred myself when choosing the title for my article.
But we should not talk about David Hoult as though he were some abstract entity. We should contact him and enquire about his own current opinion about this topic. Which is what I plan to do right away.
|
December 7, 2007
Carlos' NMR Blog
Resolution Booster
| Carlos Cobas' new blog dedicated to NMR data handling
Carlos is a young chemist, an experienced number-crunching programmer, a Company President (Mestrelab Research) who looks more like its errands-boy, an enthusiast about NMR data processing, and a friend of mine. I know that all this does not come any close to a full elucidation of his structure, but it probably gives you an idea.
He started a blog titled NMR Analysis, Processing, Prediction and Verification and dedicated to all those aspects of NMR spectroscopy software which are either essential to, or appreciated by, chemists. For what this entails in the 1D NMR case, see the presentation about Advances in Computer-Assisted Evaluation of NMR Spectra we gave at the SMASH-2007 meeting. Not that Carlos' interests cover just 1D NMR - on his blog he will certainly dedicate a lot of room also to higher-dimensional NMR data evaluation.
I admit that I have a vested interest in the new blog since I collaborate with Carlos and with the dynamic Mestrelab team on the new Mnova software. Indeed, there will be a certain division of topics between this blog and Carlos'. My own contributions to topics such as NMR spectra simulation, fitting, processing and evaluation will be mostly discussed on Carlos' blog, while here I will continue to concentrate on other aspects of Magnetic Resonance, in particular its physics and engineering.
I want to use this occasion to mention three novel data evaluation algorithms we have just developed. Since we are going to present them as posters at the 49-th ENC meeting, I give you the links to the three poster abstracts: Bayesian DOSY and ROSY Transforms, Resolution BoosterTM, and J-CorrelatorTM.
One of these has been also already discussed on Carlos' blog (the Resolution BoosterTM) and the others will soon follow. We are still working on them and temporarily holding back on the math - it is a whole new area and we want to explore it better before coming up with scientific papers.
But it will not take long, so stay in touch!
|
December 5, 2007
| Quadrature versus linear detection, and where do complex MR data come from
A couple of months ago, Alexandru Cernicanu has mailed me the following query pertinent to the article K-space formulation of MRI:
I would be very grateful if you could clarify one point for me, maybe on your blog:
When we do not use quadrature detection, how can we obtain a complex k-space?
A real object that is imaged will always have a complex k-space with complex conjugate symmetry. What happens when we do linear detection? Our k-space is not complex? How can we get back the image then?
I have seen several times the exercise where we mix the phase of a k-space from one image with the magnitude of the k-space from a different image, and people conclude triumphantly that its the phase of the k-space that matters most. That is, it is not so much the amplitude of the spatial frequencies of the image that "make" the image, but the way these spatial frequencies are dephased with respect to each other and generate spatial interference patterns.
If it is the case, we always need the k-space phase information, otherwise there is no image after the FFT? And if it is so, then it means we always have to do quadrature detection. Then how come there are still linear receive coils?
In a lot of texts they just say "quadrature detection is used for the sqr(2) SNR improvement", and don't say a word about the k-space being complex ... I am a bit confused. Could you please tell me where the fallacy is in these arguments, there is definitely something i am missing.
Dear Alexandru, you are definitely not alone to be confused. The way complex numbers arise from a real word - and the way they achieve the importance they have - is a very universal, non-trivial topic. The reason I am so late answering your query is that I wanted to write a full article about it but could not find the time to do it yet. So, for the time being, let me try just a brief answer.
First of all, it is absolutely correct that the complex phases of the k-space signals encode the essence of the final MRI image (the locations, orientation and boundaries of its constituent parts) while the k-space magnitudes affect "only" image intensities, contrast, etc. The mathematical reason behind this is evident from Eq.(14) of my article which describes the effect of an object's translation and from a similar expression describing the effects of a rotation. In the case of a translation, for example, the k-space image of the object is multiplied by a unary complex function of the k vector, i.e., one which has everywhere the same magnitude of 1 but a k-dependent azimuth (phase). There is a close parallel between this and the properties and manipulation of JPEG images (photos). There one also exploits the inverse space and uses algorithms similar to what we do in MRI to modify certain non-essential aspects of an image (luminosity, contrast, brilliance, saturation) while leaving invariant its essence (an elephant remains to be an elephant). The latter could only be changed by morphing techniques which do affect k-space phases. That, however, is something we most definitely do not want to do in MRI.
The answer to your query lies in the fact that the two Cartesian components (real and imaginary) of the k-space signal are not independent. When, as is the case of MRI, the investigated system behaves linearly, the two components are mutually related by what in some fields is known as Kronig-Krammers relations (KK) and what amounts mathematically to a Hilbert transform (HT). In classical spectroscopy, KK relations consist in a 1D HT, while in MRI they become a 2D or 3D HT, but the principle is the same: knowing one Cartesian component, one can compute the other and vice versa.
Even more is true: Suppose that you first take a single detection device (what you call a linear-coil) and acquire a single array of real data S(t). Then you take a quadrature detection device (such as an orthogonal pair of coils A and B) and acquire two arrays of data A(t) and B(t). Now, apply real Fourier Transform (FT) to S(t) to get a complex spectrum su(ω)+j.sv(ω); su and sv being the cosine and sine transforms. Likewise, apply complex FT to the complex (quadrature) data set A(t)+j.B(t) to obtain the spectrum qu(ω)+j.qv(ω). You will find out that, up to a constant phase shift and a constant magnitude factor, su(ω) = qu(ω) and sv(ω) = qv(ω). This is not something automatic: it is a theorem valid only for linear systems (linear in terms of their response to an excitation), and it belongs to the same category as the KK relations. In such systems the complex data may arise either "directly", from a hardware containing two mutually orthogonal detectors, of "indirectly", through mathematical manipulation of the data obtained from a single detector. The theorem guarantees that the two results are fully equivalent.
I am sure that you already see how this answers your query. In MRI we do not have the frequency ω characteristic of spectroscopy, but the vector k. This, however, does not change the above discussion which is independent of ω. Every linear, harmonic transform of the time-domain signals simply gives rise to a specific variation of the theorem.
What may still be not so clear is the question of sensitivity.
In the case of a single-coil detector, the noise in su and sv looks different but actually is not (one can easily test that the noise in one can be exactly reproduced by the HT of the noise in the other). The sensitivity gain is linked to the fact that, when the radio-frequency of the signals is down-scaled relative to a reference frequency Ω, the latter can be made to coincide with the center of the needed spectral window. Having both su and sv (the old-time quad detection of NMR spectroscopy) rather than just one (the old-time single detection) one can halve the cutoff frequency of the audio output filters and thus gain a factor of sqr(2) in SNR. In this case, the SNR gain is not in the signals themselves - on that side, the difference is only whether we do just a cosine transform, or both cosine and sine transforms. The difference comes from the audio filter settings and has to do with the fact that, having both su and sv, we can distinguish the signs of frequencies relative to Ω (note that Ω is close to the Larmor frequency of the observed nuclides).
When we use two orthogonal detection coils A and B, the noise in qu is independent of that in qv. This leads to a sensitivity gain of sqr(2) due to the presence of two independent detection devices. Each of the two signals A(t) and B(t) can be in fact evaluated using the old-time quadrature approach, the resulting spectrum of k-space signal from B phase-shifted by 90 degrees, and summed together with that of A. Incidentally, this again allows us to place Ω in the center of the spectral window, halve the width of the audio filters, and thus achieve a combined gain of 2 in SNR. From the electronic point of view, one could also say that one of the two sqr(2) factors comes from distinguishing (and rejecting) the band around -Ω from that around +Ω. Two orthogonal coils amount to a chiral detector which can distinguish the absolute frequency, including its sign (for example, decide the sign of the gyromagnetic ratio of the observed nuclides). No other way can achieve this (for related matters, click here).
I hope that this answers your questions. Anyway, I promise to write an illustrated article to make all this more explicit.
|
November 19, 2007
Some γ values
in MHz/Tesla :
Electron:
-28024.953
Positron:
+28024.953
Muon μ-:
-135.539
Muon μ+:
+135.539
Triton 3H:
+45.415
Proton 1H:
+42.577
Helion 3He:
-32.434
Neutron 1n
-29.165
Deuteron 2H:
+6.536
| Is it correct to say Proton NMR ?
After reading the preceding entry, my friend Santiago Dominguez, the CEO of Mestrelab, has mailed me the following query:
I wonder what you make of the commonly referred to 'proton NMR', meaning 1H?
Knowing Santi, I suspect a little bit of leg-pulling behind the question, by my answer is serious: proton NMR makes me perfectly happy since proton is a nucleon and thus a special nuclide (yes, 1H). For the same reason, neutron NMR is also legitimate, even though neutron is not a nucleus of any chemically viable atom. Neutron has a strong magnetic moment which was measured by resonance techniques already in the 40's (click here and search for neutron). The term neutron NMR was used occasionally in Russian literature, while western sources refer more often to neutron spin resonance. Since thermal neutrons gas is today a rather common substance (never mind that chemists do not like it), I suggest that in the systematic NMR nomenclature we denote it as 1n NMR.
I guess that in some distant future we will also have antiproton NMR, antineutron NMR and, in general, anti-nuclide NMR. In the systematic nomenclature, we could distinguish these by making the nucleon number index negative, like -1H NMR, -1n NMR or -13C NMR.
All other nuclides with special names are of course also legitimate NMR candidates. This encompasses deuteron NMR (2H or 2D), triton NMR (3H) and helion NMR (3He). It is also admissible to say deuterium NMR and tritium NMR because these names refer to atoms with a specific nuclide, while helium does not - a helium atom can have as its nucleus either a helion (3He) or an alpha particle (4He). Somebody could object that since helium has only one isotope whose nucleus has a non-zero magnetic moment, saying helium NMR is specific enough to avoid confusion. The same argument would than apply for many other chemical elements (like P, O, F, Al, ...). I see the point but object to it anyway, because it is not universal (for example, it does not hold for N, B, Cl, Xe, ...) and it presumes an a-priori knowledge not embodied in the name.
Then there is a group of lepton spin resonances but, since leptons are no nucleons, these can not be classified as NMR. The family includes the flourishing electron spin resonance (ESR), the well established muon spin resonance (μSR), and the unheard-of positron spin resonance. The electron resonance crowd has been discussing since years whether the equivalent terms ESR and EPR (electron paramagnetic resonance) should be both replaced by electron magnetic resonance (EMR); the result is, so far, that all three terms are being used interchangeably.
In principle, we should also consider the intermediate group of mesons (pions, kaons and eta-meson) but they have no spin and therefore no magnetic moments, and the group of heavier-than-nucleons hyperons (lambda, sigma, xi and omega). Hyperons have spins and magnetic moments but, again, their magnetic resonances can not be classified as NMR because they are no nuclides. Moreover, they are experimentally impossible to tackle since they live only a fraction of a nanosecond.
|
November 15, 2007
1H-NMR of
CH3CH2OH:
Some isotopomers:
12C1H312C1H216O1H
System: A3B2C
13C1H312C1H216O1H
System: XA3B2C
12C1H312C1H2H16O1H
System: A3BXC
Isotopomer counts:
C: 12C, 13C
2x2 = 4 cases
O: 16O, 17O, 18O
3 cases,
2 magn.distinct
H: H, D
2 cases
H2: H2, HD, D2
3 cases
H3: H3, H2D, HD2, D3
4 cases
Totals:
4x3x2x3x2x2 = 288
Magnetically distinct:
4x2x2x3x2x2 = 192
Al least one proton:
4x2x(2x3x4-1) = 184
etc.
| Nuclides versus nuclei
Some of my friends noticed my tendency to use the term nuclide instead of nucleus and wondered whether I do so to make myself more interesting or whether there is something deeper in it. I do not deny that their worst suspicions may be partly correct since, as a physicist, I need to distinguish myself from the more abundant chemists :-)
Speaking more seriously, I do think that the term atomic nucleus is a bit too generic (it somehow recalls the times when Lord Rutherford was scattering alpha-particles on gold foliums). What resonates in NMR are no generic nuclei, but very specific nuclides. We have 13C-NMR but no 12C-NMR, and we have 1H-NMR which is quite different from 2H-NMR. So, the way I see it, terms like hydrogen NMR or carbon NMR should not exist. They are in current use, but they indicate something much narrower than what they say. By the same reasoning, even the present term Nuclear Magnetic Resonance should be replaced by something like Magnetic Resonance of Nuclides (MRN).
Using the chemical name of an element in a nuclear context, one tends to disregard isotopic composition. A term like HNMR can therefore open the door to potential confusion simply because no substance is isotopically pure. For example, even a molecule as small and simple as ethanol (CH3CH2OH) has 288 isotopic varieties, 192 of which magnetically distinct.
These give rise to 184 1H spectra, 184 2H spectra, 144 13C spectra and 96 17O spectra, all nontrivial and, in principle, distinct from each other! It is true that the least abundant of these isotopomers is so rare in nature that you would need over 100 moles of ethanol to have any chance to meet one of its molecules, but that can change completely when one starts playing with isotopic enrichment (like when NMR is used to elucidate metabolic pathways). Or when one focuses on just a few most abundant isotopomers, like in SNIF-NMR (Site-Specific Natural Isotope Fractionation). I am also convinced that spectral lines of trace isotopomers, such as the 13C satellites in 1H-NMR spectra, will become an important routine tool for structure verification and elucidation.
So when somebody asks me to simulate the hydrogen NMR spectrum of ethanol, I cannot but ask: do you mean the natural-abundance mixture or a particular isotopomer? And I will continue using the term nuclides in my own texts, though I am really not as fussy about it as I sound. However, if you have any counter-argument in favor of nuclei, let me know and I will post it.
|
November 3, 2007
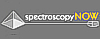
| Welcome to SpectroscopyNOW readers!
SpectroscopyNOW started live channels showing leads to this blog's five most recent items on their NMR and MRI main pages. This is an unexpected honor showing that I am possibly doing something right. In a sense, it is also a liability since I will be now bound to stay on the virtuous path, no matter what! Thanks, SpectroscopyNOW, and let the readers' click-fingers decide whether you do not overestimate me.
Allow me to use this occasion to mention that visits to this site started crossing the 1000-a-day mark. Thank you all! Though I am right now behind with answering a couple of queries and publishing an interview or two, I will do my best to stay abreast. And, please, keep letting me know about NMR/MRI/ESR/NQR events you might be organizing, books you might have written, and Companies you might have set-up or revved-up.
|
October 30, 2007
Antenna Theorem Summary
Sakellariou MACS Rotor:

Yellow: capillary
Brown: microcoil B
Red: cap to tune B
Green: 7mm fitting
Blue: standard rotor
Ochre: outer coil A
1H performance 0.41mg L-alanine:
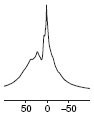
Standard rotor:
Rotor diam: 2.5 mm
Accumulation: 12 h
Scale: ppm
Strong background

Standard rotor minus background:
Total accum: 24 h
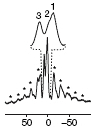
Sakellariou rotor:
Total accum: 8.5 min
No background
The four Figures
above were adapted
from reference [1]
| MACS: The magic of inductive coupling in MAS spectroscopy
The fact that mutual induction between coils can be used for a contact-less detection of nuclear magnetic resonance signals was obvious since the very start of NMR. For a long time, however, it did not attract too much attention since it was viewed, at best, as a trick to alleviate the congestion of the sample area, rather than something that might boost the sensitivity. The situation has changed after David I.Hoult has shown that the nuclear induction experiment has actually little to do with classical induction and a lot with RF antennas and the way magnetic dipoles interact with the magnetic component of their electromagnetic fields. The adaptation of the antenna reciprocity theorem to magnetic resonance, and the realization of its key role, has paved the way towards more sensitive front-end designs for MRI.
Such devices exploit the fact that a relatively small coil B, coupled inductively to a larger coil A, modifies the RF field generated by the latter, boosting it inside B by a factor f > 1. According to the NMR antenna theorem, this boosts by the same factor f the sensitivity of the compound device to nuclides located inside B (see the illustration in the left column). In a sense, the high sensitivity detector becomes the inner coil B which relays the detected signal by means of classical induction to the outer coil A.
Strangely enough, the awareness of the antenna theorem and its exploitation in 'classical' NMR was so far slower than in MRI. Since about a year, however, we hear from various sources about a new breakthrough along this line in MAS (Magic Angle Spinning) solid-state spectroscopy. The development is due to Dimitrios Sakellariou and his coworkers at Laboratoire de Structure et Dynamique par Résonance Magnétique of CEA (French Atomic Energy Commission, Sacley, France). It is based on the realization that induction between two solenoids works even when one of them (coil A) is static and the other one (coil B) rotates about their common axis. Hence, in MAS, the inner coil B can be wound around a sample and rotated together with it about the magic-angle axis, while the standard outer coil A becomes a fixed relay station for the detected signal.
The arrangement is particularly useful when the sample, contained in a capillary, is inserted into a tightly fitting coil lodged inside a standard MAS rotor. The result is a MACS microcoil rotor (MACS stands for Magic Angle Coil Spinning) with excellent sensitivity! There is also an additional benefit. In standard NMR spectroscopy, microcoils tend to locally destroy field homogeneity due to susceptiblity effects (there are various techniques to combat this but none is free of problems). In the Sakellariou rotor, this issue does not exist since such inhomogeneity broadening gets removed by the magic angle rotation.
Last July I have contacted Dimitris with a request for reprints of his papers and asked him a few questions which he promptly answered. The exchange amounted to a mini-interview which I somewhat belatedly make public:
Dear Dr.Sykora,
Thank you for your email. I will try to answer your questions point by point :
Question: Is it correct to say that the MACS development is "all French"? Or is Alex Pines' group involved somehow?
Answer: Yes, it is a project that was conceived and realized after my recent return three years ago in Europe (in the CEA). The Pines lab is not involved with this project for the moment. What deserves to be said is that MACS corresponds to an "out of the box" idea, typical of what has been going on for many years in Berkeley. I spent 4 years of my life there, so I want to believe that this might have changed a bit my way of thinking, and for that I am deeply grateful to Alex Pines.
Question: If I get it right, you wind the microcoils yourself. This is probably not easy - both the winding and the choice of wire and of the tuning capacitor. Could you comment on this?
Answer: This is true, we manually wind the coils under a stereoscope, we glue them on the capillary and we use empirical formulas for the inductances in order to choose the right chip capacitor. This leads to not perfectly shaped coils, non-reproducible winding and most of the times to the need to change a capacitor, if the frequency is not close enough to the Larmor frequency.
Question: Do you think that one could manufacture rotors with a microcoil insert for exchangeable sample capillaries? It might be an interesting product for a small Company (a university spin-off), a fact I would like to mention.
Answer: Definitely this is possible and wished. We do not have the resources to do it ourselves in the CEA, but there are many approaches for making microcoils automatically, possibly integrating the tuning capacitor in the design. The final insert should have enough space for exchangeable sample capillaries and also outside fittings to fit inside various rotor diameters.
Question: From this point of view, is there any [pending] patent regarding this matter?
Answer: Yes, we have made two patent applications on the principles of the method and various practical realizations.
Many thanks and please feel free to come back with more questions if needed.
Sincerely yours, Dimitris
Dimitris is a young man and, I am sure, a great promise for European NMR. He stayed for some time in the Alex Pines group at UC Berkeley (Chemistry), and co-signed several revolutionary papers on yet another novel NMR thread: high-resolution spectroscopy in inhomogeneous fields, a project cherished by Alex Pines himself. He is now trying to set up his own group at CEA and he certainly deserves all the support he can get.
Selected References:
- Sakellariou D., Le Goff G., Jacquinot J.-F.,
High-resolution, high-sensitivity NMR of nanolitre anisotropic samples by coil spinning,
Nature 447, 694-697 (June 2007).
doi: 10.1038/nature05897. The seminal paper on MACS.
- Edison A.S., Long J.R.,
The magic of solenoids,
Nature 447, 646-647 (June 2007). A News and Views [over]popularization.
- Hoult D.I., Tomanek B.,
Use of mutually inductive coupling in probe design,
Concepts Magn. Reson. B15, 262-285 (2002).
Review of principles and applications of inductive coupling and reciprocity.
- Hoult D.I.,
The principle of reciprocity in signal strength calculations - a mathematical guide,
Concepts Magn. Reson. 12, 173-187 (2000).
In-depth summary of the reciprocity theorem.
- Hoult D.I., Bhakar B.,
NMR signal reception: Virtual photons and coherent spontaneous emission.,
Concepts Magn. Reson. 9, 277-297 (1996).
Argumentation in favor of the reciprocity principle.
|
October 27, 2007
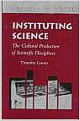
| 'Early NMR at Varian' - a clarification by Tim Lenoir
At the Stanford University server there was, time ago, an orphaned article by Timothy Lenoir and Christophe Lècuyer titled Early NMR at Varian. Though it was untended and all its links were broken, I liked it because it contained interesting reminiscences about the pioneers of NMR and a convincing and detailed review of the early commercial exploitation of NMR by Varian, written by authors outside of the NMR community but with an excellent command of the topic. Last spring, however, the article disappeared from the server. In an attempt to recover it, I wrote to Tim Lenoir and [today] received the following answer:
Dear Stan,
I am sorry it has taken so long to get in touch with you. Your letter was forwarded to me from Stanford after some time. I moved from Stanford to Duke University two years ago, and mail that arrives for me there does not get forwarded so easily. I appreciate your interest in the paper that Christophe Lecuyer and I did on NMR. That paper was published in Perspectives on Science, and a slightly revised version appeared as a chapter in my book, Instituting Science. Christophe and I would be delighted to have it included in your collection. I have created a pdf version of the publication from Perspectives, and I am attaching it here. I am also putting it on my website here at Duke, but it won't be available for a couple of days from now. In any case I am delighted to be in touch with you.
Best, Tim Lenoir
So this explains the whereabouts of the text. I have dutifully corrected all the pertinent references on my web page Works about the History of NMR and MRI and I have also checked the site of Perspectives on Science. The Journal has TOC's and electronic versions of articles starting only from Volume 6, while Tim's and Christophe's article appeared in Volume 3 (pp.276-345, 1995) under the definitive title Instrument Makers and Discipline Builders: The case of Nuclear Magnetic Resonance. This, together with the above waiver from the Authors, should make it legal for you to consult the PDF (4 MB), as long as you do so strictly for your personal use. If you want to read the revised text, the book (Instituting Science: The Cultural Production of Scientific Disciplines,
Stanford University Press, 1997) is available from Amazon.
This long article (70 pages, 8 of which are titled references) is a choice reading for those students of the NMR technique who wish to acquire a real-life feeling for the story of NMR origins. Which does not mean that other accounts are false. They just tend to be idealized, while this one makes you understand what a mess it is to bring scientific discoveries to practical fruition. It should be a compulsory reading for university spin-off managers and for anybody intending to start a high-tech Company.
|
October 22, 2007
| The ultimate size of the MRI market
From a very interesting page of EMRF (the European Magnetic Resonance Forum) I extrapolate that there are presently about 28'000 MRI scanners in the world, located mostly in the USA (>13'000), Europe (>7'000) and Japan (>5'000) and the current production is somewhere around 2'500 units/year. The number of installed scanners is now superior to that of NMR spectrometers and the respective production rates differ by a factor which, I guess, is between 4 and 5 and growing.
Most striking is the territorial distribution of the scanners. According to EMRF, the 2006 scanner-density record went to Japan with 44 imagers per million inhabitants, followed by the USA (37), Europe (11, with a peak of 18 in Norway), China (3) and India (0.5). I am sure that Australia is also pretty high, but it is not listed. Neither are Latin America and Africa (in the latter case it is likely that the number does not even reach a measurable threshold). It is hard to imagine a better example of the abyssal differences between different areas of this planet.
I have done a fast mental check on those numbers. Suppose that, on the average, a person is likely to have one MRI scan in a year (most of my Italian friends expect something of that order or better). Suppose further that each installed scanner is operated 24 hours a day for 365 days a year, scanning two patients per hour (this is totally over-optimistic, but let us pretend that it's possible). If one works out the math, this indicates the need for 57 scanners per million inhabitants. So, by these standards, Japan is close, US have passed the halfway mark, Europe has made the first steps, and much of the rest is plodding far behind, if not stuck at the start-up line. Referred to the world population of over 6.6 billion, it amounts to a total of about 400'000 required units. If these were to be delivered within this century, the average production would have to top 4'000 units/year, or nearly twice the current capacity, despite my very unfavorable assumptions! This, moreover, does not take into account replacement needs: if the average lifetime of a 2100-vintage instrument were 20 years, a production of 20'000 units/year would be needed just to maintain the installed park!
These (under)estimates should be good news for MR scanner producers, except for the fact that most of the world is too poor to afford their products. A huge but poor potential market implies an acute and unrelenting competitive pressure which will probably last for most of this century. The winners will be Companies capable of producing in large volumes reliable and long-lasting scanners at a fraction of the cost we are accustomed to today. It is either this or else the gap between the developed countries and the rest will keep growing. How can the producers make it? For one small contribution, click here.
|
October 15, 2007
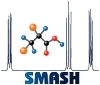
| Impressions from SMASH 2007
Although a month has already passed since I returned from the SMASH 2007 meeting in Chamonix, France, I would still like to share with you some of my impressions of the event. The conference was held at a beautiful place (see some of the photos I have taken there) but that, by itself, does not say much about its scientific value and contents.
This annual meeting concentrates on NMR of small molecules. Which, unfortunately, is a very vague definition. I kept asking around what small molecule meant and was receiving answers like these:
- Anything of interest to pharmaceutical Companies
- I feel comfortable up to 300 Daltons but I guess that it might be as high as 500
- Anything but proteins, nucleic acids, polypeptides and polymers
- At Glaxo-SmithKline we ignore anything above 250
- Anything that can be completely verified from NMR spectra
So this is something the Organizers might wish to specify better prior to SMASH 2008 which will meet September 7-10, 2008, in Santa Fe, New Mexico (I am joking, of course).
The 300 or so SMASH participants include a pool of academic people, mixed more than generously with industrial researchers, many of whom come from pharmaceutical Companies. Schematically speaking, all are very practical and passionately interested in molecular-structure problems ranging from structure verification to elucidation and interpretation of spectra of individual molecules and their mixtures. And they will hardly accept any lengthy story about an NMR puzzle solved after years of study. They want fast solutions and they want them now. They deal with hundreds of samples a day and never have enough experienced chemists to interpret them; so anything that takes more than 10 man-minutes is out. Well, I am exaggerating, of course, but you get the idea.
Also, I should not say man-minutes since the audience was very much gender-equilibrated, confirming my old impression that women really love fooling with molecules.
The practical orientation and the presence of presumably rich Companies make this meeting a favored choice for equipment manufacturers and NMR software houses (vendors, as they call them). As Paolo Santino from Varian's Italian office puts it: This is the best one! There was also an unusual number of User meetings going on in addition to the Conference program and no doubt a lot of Companies were unobtrusively spying on each other. All the major software Companies dealing with NMR spectroscopy were present and offering live demos: Mestrelab Research, ModGraph Consultants, Perch Solutions, and ACD, to name just the most important ones.
I have thoroughly enjoyed most of the talks, many of which touched upon what I consider my childhood passions like spectra simulation, fitting, and structure verification (my invited talk centered on Computer-Assisted Evaluation of 1D NMR Spectra). I used to do these things extensively in mid 60's and mid 70's, and I am returning back to them now through my collaboration with Mestrelab.
What surprised me was the fact that, to look closely, not so much happened in this area during the years my attention was focused elsewhere. My old thoughts and puzzlements are still present, pertinent, and mostly unsolved. This, no doubt, must be due to the fact that so much has happened in so many and so diverse fields of NMR that there just was no time to handle it all. There is still an awful lot to do but, at the meeting, I had a distinct feeling that this was on the minds of a lot of people. I foresee a rapid progress in a matter of months.
|
September 29, 2007

| Drugs discovery, Dubai, and 15 Nobel prize winners
Meeting fifteen Nobel laureates in the same place and having an opportunity to chat with all of them is a rare, once-in-a-life experience. Yet that is exactly what you might be able to do next February 4-7 in Dubai, United Arab Emirates, during the
1st International Conference on Drug Design and Discovery (ICDDD).
I don't believe that anybody could still wonder about the key role of NMR spectroscopy in the discovery and development of new drugs. But should you have the slightest doubt, just consider that this meeting's Organizing Committee is Chaired by no less than Richard Ernst who will also deliver a 45' opening talk.
The list of participating Nobel laureates shows how interdisciplinary is this field:
Zhores I.Alferov, physics, information and communication technology
Baruch S.Blumberg, medicine, origin and dissemination of infectious diseases
James Cronin, physics, discovery of violations of fundamental symmetry principles
Johann Deisenhofer, chemistry, structure of photosynthetic reaction centre
Richard R.Ernst, chemistry, NMR methodology
Ivar Giaever, physics, electron tunneling and superconductivity
Robert Huber, chemistry, light energy and electron transfer in biology
Klaus von Klitzing, physics, quantized Hall effect
Harold Kroto, chemistry, discovery of fullerenes
Yuan T.Lee, chemistry, dynamics of chemical elementary processes
Robert C.Richardson, physics, discovery of superfluidity
John Robert Schrieffer, physics, theory of superconductivity
Martinus J.G.Veltman, physics, electroweak interactions
Robin J.Warren, medicine, discovery of the Helicobacter pylori
Kurt Wütrich, chemistry, NMR, structure and function of biological macromolecules
It is actually hard to imagine what, for example, have electroweak interactions or CP asymmetry in common with drugs discovery and avoid the suspicion of a high-octane mundane boost. But that, I am sure, is only because I am so feeble-minded. The prominent role of Richard Ernst is a warranty that Science will eventually prevail over any superficial glamour and glitter.
If you would like to participate, hurry up, since the early-bird registration deadline is November 2 (abstracts are due December 31).
|
September 27, 2007


| An important ESR meeting and ESR/NMR jealousy
In just two days starts GIRSE 07, the first joint EPR meeting of GIRSE (Gruppo Italiano di Risonanza di Spin Elettronico) and ARPE (Association Francaise de Resonance Paramagnetique Electronique) which, in addition, marks also the 20th anniversary of the Italian ESR group.
I write this because I feel guilty and wish to apologize for having entered it only today into this site's list of MR events. Having unwittingly overlooked it, I might have confirmed the feeling of many an ESR spectroscopist that they are overlooked and overwhelmed by the much bigger NMR and MRI communities. I assure you that this was totally unintentional.
In Italy, the feeling was a source of a lengthy dispute within the original GDRM (Gruppo di Discussione di Risonanze Magnetiche, now GIDRM) which ended in 1987 with a 'divorce' between the NMR and the ESR components. Personally, I still feel that this was unfortunate and I notice that it left scars which are still visible. In fact, this is what I read on the GIRSE_07 home page:
GIRSE ... was born back in 1987 ... as an improbable project within a small group of Italian EPR spectroscopists who felt outcast and outnumbered within the general magnetic resonance associations they belonged to.
Although conscious of the common roots shared by EPR and NMR, the EPR spectroscopist is also aware that the two worlds obey different rules, research in the EPR field asking for scientists who are at a time skilled, crafty, open-minded, and endowed with a sound theoretical basis. These features have been through the years the basis of the strong 'liaisons' and sense of comradeship shared by EPR people, ...
There are some strong words here which, I am sure, their authors themselves found amusing (apparently most of the brutish NMR-ists can't tell a Hamiltonian from a bicycle). But, fun apart, it indicates that there still persist traces of jealousy due to the fact that, historically, NMR kind of 'stole' the stage from ESR (including the Nobel prizes).
Well, I apologize again.
|
September 1, 2007
| Who has built the first NMR locks?
NMR lock, an independent single-channel spectrometer, is an ubiquitous part of any modern NMR spectrometer, responsible for the stabilization of the magnetic field with an absolute error of about 1e-10 Tesla (nothing short of magnetic resonance can achieve such a feat). But do you know how it all started?
The idea of using NMR signal to stabilize the field of a magnet has been first published by Martin E. Packard as early as 1948 (A Proton-Controlled Magnetic Field Regulator, Rev.Sci.Instrum. 19, 435-439) who has also built the first operative unit with the reported stability of 2e-6 Tesla. It contained seven vacuum tubes - six pentodes and one dual heptode - or an equivalent of just about 28 transistors (EE guys, are you reading this?)! Afterwards, there was a long gap during which apparently no other units were built, until Saul Meiboom at Bell Laboratories has built himself an operative NMR lock around 1958-1959. In case you wonder, yes, it is the same Meiboom of the CPMG sequence.
The first commercial external NMR lock (they called it field-frequency lock) appeared on the Varian A60 instruments in 1961 - well 13 years after the first Packard's unit. Compare this with the idea of a sample spinner which was suggested by Felix Bloch in April 1954 (Line-Narrowing by Macroscopic Motion, Phys.Rev. 94, 496-497) and reached production at Varian in December of the same year. Clearly, the NMR lock was more difficult to digest.
The idea of an internal lock, due to Weston A. Anderson, has been set forth in November 1962 (Applications of Modulation Techniques to High Resolution Nuclear Magnetic Resonance Spectrometers, Rev.Sci.Instruments 33, 1160-1166) but reached the market only in 1964 (Varian). It was Jeol, an NMR newcomer, to offer in 1966 both an external and an internal lock on the same instrument (C60H). Another newcomer, Bruker Magnetics, followed the same route on their first instrument (HF60) in 1968.
If you are puzzled by Bell Laboratories having an NMR instrument so early in the game, consider another fact: the very first three commercial NMR spectrometers, all produced by Varian, were sold in 1952 to Humble Oil, Shell Development Corporation, and Du Pont - all industries !!
You can read most of this - and much more - in a book by Eric von Hippel, The Sources of Innovation, pages 143 - 153, which can be freely downloaded in the PDF format. For the URL, see the Online NMR & MRI books. If, instead, you want to buy it from Amazon, click on the image on the left.
|
August 27, 2007
DEAD-TIME illustration:
Sample: Doped water
Rx-Tx isolation: Simple Lowe-Tarr
Frequency: 21.4 MHz
Shown: FID portion
Sampling rate: 5 MHz
Hor.scale: 0 - 40 μs
Ver.scale: arbitrary
Red trace: U (real)
Green trace: V (imag)
Black trace: Modulus
Dead time: 8 μs
Preamplifier gain varies up to: 30 μs
Conclusions: Good probe Bad preamp
Multiple λ/4: Could help
| A contribution to the eternal fight against dead-time
I have put on-line an article describing a dead-time reduction trick which I had been occasionally using since years. It is a straightforward improvisation on the classical Lowe-Tarr scheme which can sometimes save the day - especially when you are doing relaxometry of systems containing solid components whose FID's decay to zero in a matter of 10-30 μs. Being simple and economic, the trick is probably far from original. Nor does it always help, since it does not eliminate artifacts due to the tails of probe or transmitter ringing which are below the crossed-diodes threshold (0.65 V) but above the preamplifier saturation level (this depends upon the preamplifier). But it does help a lot when dead-time is dominated by the receiver recovery time from a severe saturation.
What made me think about this was a comment by a friend at University of Pavia who claimed that their dead time (at about 20 MHz operating frequency) was between 1 and 2 μs and that they managed to keep is so low by simply replacing the λ/4 coaxial receiver-isolation cable with something like 5λ/4. Since I was incredulous, he proceeded to give me a practical demo and, as usual, the demo failed. His dead-time stayed at about 11 μs with both cables. The episode set me thinking until it dawned on me that (i) there was something to my friend's multiple λ/4 trick but (ii) it needed a little refinement. I have then tried it many times and found it useful in about half the cases (sometimes spectacularly so), depending upon which dead-time factor was dominant.
Original or not, the simple trick merited to be written down and shared with others. I have also collected over 20 titled references dedicated to the transmitter-receiver isolation problem, starting a small, dedicated collection which should keep growing in the future.
For those who are not "in", dead-time is the period after an excitation RF pulse during which the receiver (particularly its first stage, the preamplifier) is blinded and its output signal is either nonsensical or severely distorted. An RF pulse may have a peak-to-peak amplitude of several hundreds of volts, while the peak-to-peak voltage of the subsequent FID signal may be somewhere between 1μV and 1 mV. Since the transmitter and the receiver branches meet at the common detection coil, the transition from excitation to detection can not be instantaneous. After a pulse, it takes time before the receiver becomes again operative - a time which is particularly critical when the duration of the transient FID signal is of the same order of magnitude. In any case, the dead-time should be kept as short as possible.
The last para of the article contains a safety warning which all pulsed NMR operators should be aware of. It says: Make sure that you never apply to the system high-power RF with a grossly incorrect frequency. If you do so ... you soon either burn the transmitter or burn the diodes! What this means in practice is: Don't fool with the spectrometer frequency parameter, no matter how easy it may be to change it. When you do change it, make sure that you first insert the proper λ/4 cable (or the correct lumped-circuit plug-in if your instrument uses those), tune the probe, and only then hit the GO button. Especially if you use long pulses (T1ρ measurements) or long trains of pulses (CPMG pulse sequence sections).
Another practical comment: Damaged front-end crossed-diodes may be quite a nuisance since the instrument may appear to be fully functional but have a degraded sensitivity. I remember a case when it took several service visits (and several kEuros) before it turned out that the only problem was a pair of damaged diodes costing about 1 Euro both!
|
August 23, 2007
| NMR Logging - a fine book made available online for free
Halliburton Energy Services (Houston, Texas) have made publicly available a very nice book written in 1999 by their foremost NMR experts, George R.Coates, Lizhi Xiao, and Manfred G.Prammer. Its title is NMR Logging: Principles and Applications and it can be downloaded from this page (or try the link associated with the Figure).
There are many NMR specialists who are not even aware of NMR well-logging in petrology and geological research. In some circles, the very idea of sending a huge NMR device down a kilometers deep hole and doing NMR of the rocks along the way causes hilarity. This book shows in a most convincing way that such hilarity is misplaced. NMR well-logging is today a mature discipline of considerable scientific and technical complexity and economic impact.
Though the book addresses all aspects of the topic and analyses them in considerable detail on over 250 pages, it is very readable (it is also extremely well illustrated). It builds on decades of practical experience within the NUMAR Company (now part of Halliburton) but the presentation level meets the finest academic standards.
|
August 5, 2007
| The puzzling FDA limits on dB/dt in clinical MRI
I have noticed the following sentence in Joseph Hornak's article (see previous entry):
In the USA, the maximum dB/dt from a gradient pulse is now limited by the value that causes nerve stimulation in the patient.
Puzzled by such an uncharacteristic FDA lassitude, I have checked with Joseph to make sure that there was no error. This is his reply:
The answer to your question about current US FDA limits on dB/dt is:
As long as there is no discomfort, pain, or nerve stimulation, you can do it.
The reference is: Criteria for Significant Risk Investigations of Magnetic Resonance Diagnostic Devices, July 14, 2003, U.S. Department of Health and Human Services, Food and Drug Administration, Document 793.
This is truly puzzling. The document is available on internet and the actual wording it uses borders on the incredible. For 'gradient fields? it excludes only 'any time rate of change of gradient fields (dB/dt) sufficient to produce severe discomfort or painful nerve stimulation'. In other words, anything less than outright torture is acceptable!
There must be some misunderstanding here - especially since European regulations are extremely stringent. I intend to write to the FDA contact person to find out what is amiss (stay in tune).
|
July 27, 2007
| MRI Hardware: A two decade retrospective
Joseph Hornak, the author of the popular on-line books The Basics of NMR and The Basics of MRI, send me an interesting reflection prompted, to some extent, by my blog entry about the Impending NMR/MRI hardware revolution. Compared with the latter, however, his point-of-view is more historic and his perspective covers the whole routine MRI scanner rather than just its digital parts. In fact, he starts by saying:
Over the past 23 years I have seen many changes in the hardware used on MRI systems.
I summarize them below from the perspective of walking up to an MRI scanner for a scan.
I thought that Joseph's text was a bit too long for a blog entry and also that its quality merited a more permanent exposure. I have therefore published it as an article in Stan's Library. It is an easy, descriptive reading which I recommend to everybody: experts are likely to become nostalgic over it, while students and even would-be patients will effortlessly enrich their understanding of the MRI technique.
These online texts by M.S.Cohen illustrate some points mentioned in Joseph's article:
Echo-planar imaging (EPI) and functional MRI.
Speed limit in MRI.
|
July 24, 2007
CONTENTS & PREFACE
| A new book concerned with teaching NMR spectroscopy
This book, edited by David Rovnyak and Robert A.Stockland, is the first one of a kind and should have an enormous success (see its Contents and Preface).
There are quite a few books dealing with NMR spectroscopy on an introductory level suitable for neophytes and students. However, it is one thing to explain a subject in a simple way (no easy task by itself) and another one to devise and describe the best ways to teach it. In addition, there is a third aspect, that of exploiting a subject for teaching in general. As far as I can tell, while the first of these three themes is relatively well covered in NMR, the other two are not and this book may be an advance sentry in this area.
To think about it, it is a bit strange. There are mountains of books about teaching math (and also about how to exploit math in education). There are also some books (not many) on how to teach physics. But in several other fields, such as NMR and electronics, I am not aware about many such texts, despite their obvious usefulness.
|
July 17, 2007
Record Magnetic Fields
Peak 2800 T explosion
Appreciable duration 850 T / ~8 μs explosion
Pulsed, reusable 70 T / ~5 ms Bitter type
Steady 45 T / 30 MW hybrid
Supercons 23 T / 0 W
Permanent 5 T / 0 W rare-earths
Classical electromagnets 2.3 T / 10 kW iron-group
| The peak magnetic field ever reached in the Sol System
Hardly a day elapses between when I publish an article and when I am rebuked !!! :-)
In an email, Andrey Sidorenko draws my attention to the fact that the highest achieved field is >20 MG and adds the reference listed below.
I have purchased the article and found out that:
- the highest magnetic field reached so far in this Solar system is about 2800 T,
- the mark was hit at the RFNC-VNIIEF facility in Sarov, Russia,
- it takes sophisticated multi-stage explosive devices to produce the fields,
- their 'sample' diameters are about 4 mm, and
- there is a whole international network of MegaGauss research.
The magagauss guys are concerned with plasma physics, nuclear fusion theories, and extreme states of matter. They do not do any NMR or ESR, though I think that it might be both feasible and useful for precise field intensity measurements. During the field build-up from the starting 12 T or so to the final 2800 T, excited protons, for example, would produce a variable-frequency signal peaking at 119 GHz which might be detectable by remote antennae (a kind of NMR screech).
I could not amend my state-of-the-art diagram because, unlike the DIRAC experiment field of 850 T which reaches a plateau, the more recent field records can not be associated with any measurable duration. The field simply keeps growing until the whole thing is destroyed. The field measurements are optical and the measured values are quite uncertain (they have calibration problems). According to the diagram, the field should have lasted some 700 ns, but this is not born out by the published data. Consequently, I have just 'corrected' the previous blog entry by introducing the term magnetic field of appreciable-duration.
Note: One thing that irks me is that the magagauss crowd - just like the astronomers - measure magnetic fields in Gauss (0.0001 Tesla) which is a unit NEITHER recognized, NOR tolerated by the SI System of Units. How is it that physicists first make a big fuss about international units, force everybody to go metric, and then themselves use something else ! It is a shame !!!
The reference is
- More then 20 MG magnetic field generation in the cascade magnetocumulative MC-1 generator, by B.A.Boyko, A.I.Bykov, M.I.Dolotenko, N.P.Kolokolchikov, I.M.Markevtsev, O.M.Tatsenko and A.M.Shuvalov, in Megagauss Magnetic Field Generation, its Applications to Science and Ultra-High Pulsed-Power Technology, World Scientific Publishing Co (proceedings of the VIIIth International Conference on MegaGauss Magnetic Field Generation and Related Topics, Tallahassee, FL, USA, October 18-23, 1998).
|
July 15, 2007
June 24, 1997,
Los Alamos.
Field: 850 T
(36.2 GHz for 1H)
Duration: 8 μs
| Man-made magnetic fields: a state-of-the-art diagram
In my last entry on this topic, I said that the highest man-made fields achieved so far by self-destructive, pulsed devices were "over 100 Tesla". Following up on a presentation by Luca Bottura, I realized that this statement was somewhat misleading. The highest field of appreciable duration generated so far was 850 Tesla, achieved on June 24, 1997, at Los Alamos National Laboratory. The field lasted about 8 microseconds before the device was destroyed in a carefully planned explosion (photo on the left).
The error has prompted me to pay more attention to the duration of the field-generation event and try to produce a state-of-the-art diagram which relates the maximum achievable field to its duration (time interval for which it can be sustained) and also to the required peak power. The diagram regards devices generating magnetic fields in volumes of a few cubic centimeters and is very approximate, based on few actual data and some crude estimates. In particular, the power (be it a continuous or a peak value) may be several times lower or higher than in an actual device, depending upon the employed technology and upon the desired field parameters (such as homogeneity). Even so, I find it quite interesting:
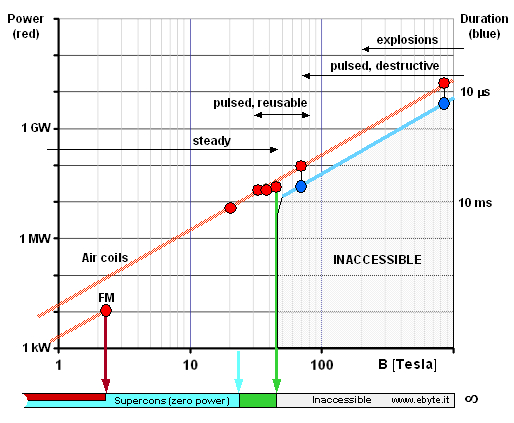
Starting from the lower left corner, we have first the area of magnets based on ferromagnetic materials (FM, brown). Here the power consumption curve regards traditional NMR-class electromagnets (for permanent magnets the power consumption is null). This technology has an upper limit of about 2.3 T and unlimited field durations. The latter fact is expressed by mapping the region onto the unlimited-duration bar at the bottom (on the logarithmic duration scale, the bar would be the 'infinity horizon').
Without the help of ferromagnetic materials, generation of magnetic fields takes about ten times more power, as indicated by the red line for resistive coils. The required power is proportional to the square of the field, so the log-log slope of the red line is 2. The actual required power depends upon many factors (choice of material, magnet type, cooling efficiency, etc). To give you an idea, a modest 20 T Bitter magnet needs almost 6 MW of power when field homogeneity is of no concern but might need over 10 MW otherwise.
Considering that NMR spectroscopy is today done almost exclusively between 5 and 23 T, this makes us appreciate how extremely lucky we are that superconductivity has been discovered (H. Kamerlingh Onnes, 1911). This genuinely quantum phenomenon makes all the difference between tens of MegaWatt and zero Watt! On the unlimited-duration bar, the region of superconducting magnets is colored light blue. For reasons discussed elsewhere, it is unlikely that it might get extended beyond 23 T any soon.
Moving further to the right we have a narrow interval (23 - 45 T) where one can build plain Bitter magnets or hybrid magnets (combination of supercons and Bitter magnets) which can sustain the field indefinitely (green area on the unlimited-duration bar). Currently, magnets in this category are publicly accessible at several high magnetic field laboratories such as the NHMFL (Tallahassee, Florida), HFML (Nijmegen, Netherlands), and TML (Tsukuba, Japan). The maximum fields generated by their non-destructive devices are about 45 T, 32 T, and 38 T, respectively, and the required powers are well over 30 MW. The steady-field magnets at these facilities are used routinely for NMR experiments, including spectroscopy and even magic-angle spinning techniques.
From 45 T up, unlimited-duration fields can not be generated because there are presently no known materials which might keep the magnets from imploding. Up to about 70 T, however, one can construct magnets which resist without damage for times of the order of several milliseconds (re-usable, pulsed magnets). The highest-field NMR signal ever measured (a deuterium FID at 58 T) was acquired during one such "shot" at the Laboratory for pulsed high magnetic fields in Dresden (Germany).
Above about 70 T the shots become destructive and the durations of the high-field pulses become progressively shorter. I do not know how many such experiments had been carried out so far. The number is probably fairly large but, as far as I could find out, none of them involved NMR experiments.
The record appreciable-duration field of 850 T was achieved on June 24, 1997 during the experiment code-named DIRAC carried out at Los Alamos National Laboratory (New Mexico, USA) in the framework of an international collaboration involving USA, Russia, Japan, Australia, Belgium and Germany. It lasted almost 8 microseconds and involved a high-power electromagnet built at the Russian RFNC-VNIIEF which was energized and immediately afterwards squeezed by surrounding explosive charges (see the photo in the left column). I estimate that the 'useful' portion of the employed peak power was well in excess of 10 GW!
The idea of boosting up a field by "squeezing" an energized magnet might have been borrowed from astronomy. Astronomers assume that magnetars with their incredibly strong fields (up to 100 GT) are imploding pulsars in which the pulsar 'normal' magnetic field (up to 100 MT) is boosted up by being squeezed (please, don't ask me what is it that collapses in a pulsar, nor what does it collapse to). Like DIRAC, magnetars are very short-lived objects - they last just about 10000 years which is the astronomical equivalent of an Earthly handful of microseconds.
Selected References and Links:
- NHMFL, the National High Magnetic Fields Laboratory with main facilities in Tallahassee, Florida, and another lab at LANL (Los Alamos National Laboratory) in New Mexico.
They detain the records for steady (45 T) and appreciable-duration (850 T) magnetic fields.
- EuroMagNET, a European consortium concerning research in high magnetic fields.
Links to six European centers working in the field, reports, meetings, calls for proposals, etc.
- RFNC-VNIIEF, the Russian Federal Nuclear Center in Sarov.
They detain the record for peak magnetic fields (about 2800 T).
- TML, the Tsukuba Magnetic Laboratory at NIMS (National Institute of Materials Science).
Japanese are very active in the field.
- Megagauss Institute sponsors the
Megagauss Conferences On High Magnetic Field Science and Applications.
- Haase J., et al,
2 GHz 1H NMR in pulsed magnets,
Solid State Nuc.Magn.Reson. 27, 206-208 (2005), and references therein.
- Haase J., et al,
Nuclear magnetic resonance in pulsed high-field magnets,
Concepts Magn. Reson. 19B, 9-13 (2003).
- Crow J.E., et al,
New scientific opportunities at very high magnetic fields,
Journal of Low Temperature Physics 89, 159-167 (1992).
|
June 19, 2007
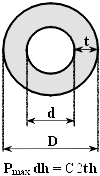
| NMR tubes under pressure and measurement units
I have noticed a blog entry on the Norell site which handles a commendable topic - that of the maximum pressure an NMR spectroscopy sample tube can withstand - but does so in a fashion which is a bit amusing and imprecise.
The funny thing is that they express the tube dimensions (wall thickness t and outer diameter D) in millimeters, which is an SI unit, and the pressure in psi (pound-force per square inch), which is an imperial unit. As a US Company, Norell should be aware that by promoting the imperial units, they are sabotaging their government's metric program! What is even worse, they mix the two systems together - a crime that should incur death penalty ... (ok, I am joking ... a life term might do :-) The fact is that until I found out that 1 psi was equal to 6894.757 Pascal, I did not know what they were talking about.
The imprecision consists in the fact that the Norell formula is overly approximate (though acceptable for thin-walled tubes). People who need high pressures are likely to opt for thick-walled tubes where it is necessary to take into account that the pressure acts on the inner walls so that the maximum allowable pressure tends to infinity when the inner diameter d tends to 0 (or t tends to D/2). The correct formula makes the maximum pressure proportional to t/d rather than t/D:
Pmax = 2C (t/d), ⇒ Psafe = κ (t/d),
where C is the tensile strength of glass (about 50 MPa or 7400 psi), κ = 2C/s, s being a safety factor. Norell has fixed the practical limit of κ at 2000 psi, equivalent to about 14 MPa. This amounts to a safety factor of over 7 - which is understandable if one considers that
(1) most countries prescribe a civilian safety factor of 5,
(2) thin-walled tubes can be easily affected by tiny scratches or irregularities,
(3) they need to protect themselves against any liability, and
(4) in thick-walled tubes the tension at the inner side is higher than at the outer side.
If you have a thick-walled tube and/or are willing to risk in the name of Science, however, you could try and push the pressure higher. It should be safe until at least κ = 50 MPa (safety factor 2) but the risk is yours, or course. Keep in mind that the tensile strength of glass increases with temperature - it is 50 MPa at about -200°C, 56 MPa at +100°C and 62 MPa at +300°C (Corning 7940). The corresponding values for quartz are almost the same.
And one more thing, in case you wonder: one atmosphere is very close to 0.1 MPa :-))
For more references on measurement units, click here.
|
June 16, 2007
| The most recent crop of MRI books
This quarter three dedicated MRI books have either left or are leaving the presses. They are all addressing clinical physicians (books dedicated to MRI physics and R&D are much less frequent). The popular Sectional Anatomy by MRI and CT is already in its 3rd edition which is quite a success in such a dynamic field. The three titles are:
- El-Khoury G.Y., Bergman R.A., Montgomery W.J., Mullan B.F., Stolpen A., Kransdorf M.J., Sectional Anatomy by MRI and CT (with CD), 3rd Edition, Churchill Livingstone.
- Mukherjee D., Rajagopalan S., CT and MR Angiography of the Peripheral Circulation: Practical Approach with Clinical Protocols, CRC Press.
- Stippich C., Clinical Functional MRI: Presurgical Functional Neuroimaging, Springer.
The last title underlines the pervasive attention being paid to the evolving methodology of functional magnetic resonance (fMRI) of the brain. It regards one of its totally non-controversial uses, but part of the growing interest is due to the fMRI potential for snooping on emotions. All these aspects had been discussed during the recent 13th Annual Meeting on Human Brain Imaging in Chicago. Those interested in the social impact of fMRI will find some pertinent info also in the book by R.L.Payne and C.L.Cooper, Emotions at Work: Theory, Research And Applications For Management (Wiley) which is slated for release in a few days.
|
June 14, 2007
These chips can hold
three NMR's each!
Images reduced 3:1
Stelar SpinWhip
board in Dec.2004.
Based on a single
Virtex II Pro, it
holds a broadband
NMR console.
| Impending NMR/MRI hardware revolution
During the last few years we have seen the big NMR manufacturers and some of the small ones (like Stelar, Tecmag or SpinCore) go completely digital - which means that they have implemented digital receivers, digital RF generators and digital gradient waveforms, in addition to all control circuitry (such as the pulser) which used to be digital already since a long time. Most of this in-house development never becomes public, so whatever one reads in Journals like Review of Scientific Instruments [3,7] appears hopelessly outdated to those who developed the currently marketed gear (the role of academy in this process would merit a dedicated article).
When it comes to prices, confusion reigns supreme. In NMR spectroscopy, the market apparently accepted the rationale that "digital is better" (true on many counts, but not a-priori) and therefore "it should cost more" (this is ridiculous, since it costs an order of magnitude less to produce). In MRI, one always pays something for novelties but, overall, the transition to digital had little impact on prices. In other fields, like NMR relaxometry, FFC-NMR or ex-situ NMR, the transition is often combined with price reductions and/or opening of new application areas.
So what is really going on? Let me try a fast answer.
There is a revolution in progress in digital electronics. It started a decade ago and reached relative maturity about 3 years ago (they are now moving into analog electronics!). At the heart of the process is the introduction of large-capacity, low-cost FPGA's (field-programmable gate arrays) by Companies like Altera and Xilinx. Today, the capacities of these devices reach tens of millions of logic gates on chips the size of a postmark and their retail prices range from as low as $100 to as affordable as $5000! Such programmable chips can accommodate tens of microprocessors and/or DSP's, tens of DDS (Direct Digital Synthesis) RF generators, RF quadrature down-converters, digital filters, sophisticated data-accumulation devices, event controllers, and incredible amounts of custom circuits, all running in parallel on clocks as high as 500 MHz (two years ago it was 300 MHz, so I guess that 1 GHz is just behind the corner). The revolution led to the birth of totally new concepts such as re-programmable hardware, libraries of reusable hardware-definition code (called IP cores, the IP standing for Intellectual Property), Systems on Chip (SoC), Electronic System Level (ESL) design, and Virtual Instrumentation (VI). It also reduced the development cycles of digital electronics by a factor of at least ten, and simplified enormously the development and deployment of systems produced in small series (exactly what magnetic resonance manufacturing is all about).
The development of digital electronics is nowadays almost identical to software development: circuits are designed by geek's sitting at PC workstations, pre-tested using simulator programs, uploaded into re-programmable hardware, and debugged using virtual instruments (scopes and logic analyzers) embedded into the same chip. During the whole process, the engineer never gets up from his/her chair, much less uses a solder iron. Laboratories crammed with carpentry, wires, flat cables, connectors, adaptors, power supplies, soldering stations, and old-fashioned scopes are to a large extent a matter of the past.
The revolution is now sweeping across consumer and military applications, propelled mainly by communications and image processing for TV and games. Scientific instrumentation in general, and MR in particular, seems to be considerably behind, but I have no doubt at all that during the next 20 years it will completely change its face. The revolution will also dramatically boost the performance/price ratio, making even sophisticated instruments affordable for everyone, including the underdeveloped countries.
Even now, it has already caused a dramatic drop in production costs. Roughly speaking, a cubic meter of electronics of the early 90's fits today comfortably into a single chip! Believe it or not, single chips like Virtex 4 (Xilinx) or Stratix III (Altera) can hold all the digital parts of a fully equipped, digital NMR spectrometer about three times over! Think about the manufacturing savings on all the carpentry, connectors and wiring, plus the huge drop in power consumption and the upward jump in reliability due to the elimination of thousands of physical connections!
But, as I said, all those savings and most of the new capabilities have yet to reach the market. Some reasons behind the delay are comprehensible - the change is too rapid and Companies badly need the revenue from their current designs to finance the transition. Most manufacturers are presently moving fast to exploit the cut-down production costs, but appear to be reluctant to press for new performance features due to lack of qualified personnel and/or the need to delay the obsolescence of existing products. Shortly, however, competition is bound to start playing its magic.
The new technology is eminently suited for competitive upstarts by small groups. Development boards and software tools are now mature and incredibly affordable (tools like the Xilinx 1.5 GByte WebPack can be downloaded for free). Entry costs are far below $10000 and, for once in History, a single gifted individual has a good chance to make a difference - be it a doctorate student or an ex-hacker who has just started his own Company. Potentially, one can do marvels in terms of completely novel performance features. Think about concepts like direct high-frequency sampling, multi-rate sampling, novel data accumulation modes, receiver and transmitter arrays, digital receivers integrated into probes, hand-held NMR instruments, and an infinity of other treats and tricks yet to be invented. All this requires individual genius, which puts a Big Company into exactly the same position as a Tiny Company (putting 20 average guys on a SoC project will only make you loose a lot of money, while a single brilliant person will make it fly).
Moreover, since the new hardware is handled like software (indeed, it is software), in a few years we are likely to see on the Internet a number of free or low-cost NMR IP's. With time, even IP's for full NMR and MRI consoles are likely to become widely available under GNU or Open Source licences (I would not mind contributing to this).
What it all means is that:
- First, those Companies which have not yet switched to the SoC concept may already be too late. Two or three more years and they will be wiped out of existence. The name of the game of tomorrow is a merciless "SoC or die".
- Second, we are going to see much more competition than in the past.
- Third, prices of routine instruments are bound to drop substantially. Since large Companies cannot afford such price drops without damaging their existing structures, they will be forced to implement qualitatively new performance features at an ever faster rate and hard pressed to find persons/collaborators capable of inventing and developing them (I have some doubts whether academy can supply them - not in Italy, anyway).
For User's, all this should be excellent news. As a physicist with some electronic notions, however, I would never buy a commercial instrument since today, once you know how, it is so easy to build one from scratch, and it is much more fun, too! The recipe is: for a fraction of the commercial price, buy the pieces which are either too delicate (pre-amplifiers) or too heavy (magnet, RF power amplifiers) and program the rest onto an FPGA development board! Once you start logging actual data into your PC, you can use one of the many low-cost evaluation packages to treat them. They are now available for nearly all MR areas (for spectroscopy, use MestreNova, of course :-).
Selected References:
- Meyer-Baese U.,
Digital Signal Processing with Field Programmable Gate Arrays (FPGA), 2nd Edition, Springer 2007.
- Al-Hashimi B., System on Chip: Next Generation Electronics, Iee 2006.
- Takeda K., A highly integrated FPGA-based nuclear magnetic resonance spectrometer, Rev.Sci.Instruments 78, 033103 (2007).
- Galkin A., Canina D., Sykora S., Ferrante G., Novel Approaches to the Timing of FFC-NMR experiments, Poster at 4th Conference on Field Cycling NMR Relaxometry, Torino (Italy), May 26-28, 2005.
- Canina D., Galkin A., Sykora S., Ferrante G., Novel Approaches to Signal Acquisition and Accumulation in FFC-NMR experiments, Poster at 4th Conference on Field Cycling NMR Relaxometry, Torino (Italy), May 26-28, 2005.
- Cofer R.C., Harding B.F., Rapid System Prototyping with FPGAs: Accelerating the Design Process,
Newnes 2005.
- Jie S., Qin X., Ying L., Gengying L., Home-built magnetic resonance imaging system (0.3 T) with a complete digital spectrometer, Rev.Sci.Instruments 76, 105101 (2005).
- Maxfield C., The Design Warrior's Guide to FPGAs, Newnes 2004.
- Hauck S., The Roles of FPGA's in Reprogrammable Systems, Proc.IEEE 86, 615 (1998).
- For more, see the lists of books on Electronics and DSP and search for keywords such as FPGA, SOC, System on Chip, ESL and System level.
|
June 9, 2007
| [it] Scuola Specialistica NMR di piccole molecole di interesse organico-farmaceutico
[Italian National Specialized NMR School on small molecules of farmacological interest]
Questa edizione della Scuola Nazionale di Risonanza Magnetica Nucleare avrà luogo nei giorni 3-7 settembre 2007 a Torino (c/o Fondazione per le Biotecnologie a Villa Gualino) sotto la tutela del GIDRM e del GIRM della Società Chimica Italiana.
Gli organizzatori (Stefano Chimichi et al) invitano gli interessati di aiutarli non aspettando fino all'ultimo (20 Luglio) bensì iscrivendosi il più presto possibile !!!!
|
June 8, 2007
| Relaxation rate units: [1/s] or [Hz] ?
I have read these days a couple of posters in which relaxation rates
(1) R2 = 1/T2 and R1 = 1/T1 ,
were reported in Hertz (Hz) rather than inverse seconds (1/s or s-1). Since time ago I had a discussion about this point with a friend of mine, I have formed a rather strong opinion in favor of the second convention which (fortunately for me) happens also to be the one used most frequently. Below I list my arguments in favor of the [1/s] convention, intended as a starting point for a possible discussion.
(a) Social convenience
Where there are physical alternatives, the adopted units become a matter of convention. Going against an established convention without a truly compelling reason generates just confusion and no benefits. In our case, the absolute majority of people dealing with relaxation of any kind (MR, dielectric, mechanical, etc.) use the [1/s] convention and there is no reason to question this status quo. There are better ways of distinguishing oneself from the crowd than violating perfectly reasonable conventions.
(b) Physical quantities are NOT defined by their dimensions
The statement "same dimension, same quantity" does not stand scrutiny. Sometimes, history did work in that sense (for example, the equivalence of heat and energy) but in those cases, there was nothing automatic (the equivalence of heat and energy was accepted only after a lengthy discussion between Joulites and anti-Joulites). In other cases, however, such a convergence is nowhere in sight. As an example, consider the case of torque (moment of force) and energy. Though these two quantities have the same root SI dimension, nobody would ever say that they are the same thing. To make sure, in fact, the former is usually labelled [N.m] and the latter [J]. Likewise, nobody will ever convince a radiation expert to switch from [Bq] to [Hz] even though 1 Bequerel is an euphemism for one event per second and its root dimension is [1/s].
(c) Comparison with other quantities of the [1/s] type
In my list, there are several categories of quantities whose root dimension is [1/s]. Of these, the only one which is always labelled as [Hz] is the frequency of a periodic phenomenon (the count of cycles per unit time). Even quantities closely related to frequency, such as phase drift, are expressed more often as [rad/s] or [deg/s] rather than [Hz]. But the most extensive subclass among the [1/s]-type quantities is that of relative (or log-scale) evolution rates. Given a physical quantity Q of any dimension (such as a nuclear magnetization component), its relative evolution rate (dQ/dt)/Q = d(ln(Q))/dt has always the dimension of [1/s]. NMR relaxation rates are just typical examples of this category which contains literally hundreds of entries, none of which is likely to be expressed in [Hz].
(d) Other physical distinctions
Relaxation rates are typical expressions of irreversible time-dependent phenomena, while frequencies are related to reversible periodic phenomena. This distinction is physically meaningful (think, for example, about the refocussing pulses which reverse offset effects, while nothing can ever reverse relaxation effects) and it is certainly important enough to be reflected in the dimensional units. It is true that the irreversible decay of transversal magnetization broadens spectral lines whose linewidth Δ, expressed in [Hz], becomes
(2) Δ = 1/(πT2) = R2/π .
This formula, however, involves a conversion factor π and, moreover, regards only R2.
Considering the formula for an ideal single-component NMR signal of unit intensity and frequency offset f,
(3) S(t) = exp(-R2t)exp(i2πft) = exp(i2π(f+iR2/2π)t) ,
we see that giving R the dimension of [Hz] we imply (i) its equal standing with frequency, (ii) dimensional equality between complex-conjugate pairs of quantities (something that is not universally accepted in physics), and (iii) the presence of a 2π conversion factor. In practical situations, it is not always clear whether the latter has been applied or not (moreover, it differs from the π factor in the equation for Δ). The net result is the possibility of confusion and endless misunderstandings.
As a conclusion, I strongly recommend not to use the [Hz] units for relaxation rates and stick to [1/s], with the numerical values of the rates given strictly by equations (1).
|
June 6, 2007
| Two new NMR books tackle relaxation theory and catalysis, respectively
During my visit to the 5th Conference on NMR Relaxometry (see the previous entry) I had an opportunity to give a rapid look at the book Theory of Evolution and Relaxation in Multi-Spin Systems, written by Danuta Kruk (Jagiellonian University in Krakow, Poland), and released last month by Arima Publishing. Danuta is a mathematical mind and she is very serious about NMR, so don't expect any biology or leisure behind the terms evolution and relaxation. The evolution regards spin density matrices, quantum operators and Liouville space, and the relaxation, be it perpendicular or longitudinal, is reserved strictly for nuclear magnetization. Since this book is close to what I once used to do [1,2,3], I can confirm that it is well done and covers a lot of ground. It is no beach reading, but if you want to understand a relaxation matrix - or if you need to actually calculate one - it can save you a lot of time and help you to avoid lots of mistakes.
The second book, In situ NMR Methods in Catalysis (series Topics in Current Chemistry, #276), is a collection of contributions edited and partially authored by Joachim Bargon and Lars T.Kuhn which should leave the presses this month. This is its Table of Contents:
- Giernoth R., Homogeneous Catalysis in Ionic Liquids
- Kuhn L.T., Bargon J., Transfer of Parahydrogen-Induced Hyperpolarization to Heteronuclei
- Niessen H.G., Woelk K., Investigations in Supercritical Fluids
- Greiner L., Laue S., Wöltinger J., Liese A., Continuous Asymmetric Hydrogenation
- Kuhn L.T., Bargon J., Exploiting Nuclear Spin Polarization to Investigate Free Radical Reactions via in situ NMR
|
June 5, 2007
| Glimpses from the 5-th Conference on Field Cycling NMR Relaxometry
This biennial Conference was held from May 31 to June 2, 2007, in Torino, Italy (it is one of those events which maintain a fixed location (Villa Gualino) and period (the next one will take place in May, 2009). I could not attend because I was abroad until the last day. But I did manage to make a flash appearance and collect a few personal glimpses.
Compared with the 2005 edition, this one appeared to be somewhat scaled down, probably due to the 'competition' from other meetings, like the prestigious 11th Chianti Workshop on Magnetic Resonance, held just afterwards. Since the contents of the two meetings overlap, many participants attended both of them (with a logistic support from the organizers).
The situation is an example of the fact that competitive overcrowding of the NMR events agenda might be becoming a real problem. This year there are at least 41 MR events just in May and June - and most of them are international!
Judging from the abstracts and posters, the 5th FFC-NMR Conference touched again on all traditional aspects of Variable Field MR Relaxometry (VF-MRR) such as the dynamics of large biological macromolecules and meso-scale structures, polymers, liquid crystals, nano-particles, and paramagnetic complexes (especially those which might be used as contrast agents in MRI). Some contributions introduced very empirical applications (such as fuel cell membranes or crude oil characterization) while, for good balance, some others were almost pure math (such as one by Danuta Kruk on competition between relaxation and polarization transfer processes). For details, download the full program.
I have enjoyed reading a poster signed jointly by the group of Christian Griesinger (Uni Göttingen, Germany) and Bruker. It regards the development of a fast-field-cycling accessory for standard supercon spectrometers which should combine fast-field-cycling with high-resolution signal detection. They use a pneumatic sample-shuffling device similar to the one build by Robert Bryant and they are presently testing the reproducibility of 1H and 13C spectra acquired after repeted shuffling of the sample out-and-in the observe field of 600 MHz. I want to return to this in a separate entry.
My name appeared on a Stelar poster entitled "Measurement of spin-spin relaxation time T2 at very low magnetic field by means of the Fast Field Cycling NMR method" (presented also at the last ENC meeting). It illustrates the considerable advances in the field stability and reproducibility of Stelar FFC relaxometers. It is now possible to (a) pre-polarize a sample, (b) switch to the observe field and apply a 90° pulse, (c) switch to a relaxation field and stay there for a time of τ/2, (d) switch back to the observe field and apply a 180° pulse, (e) switch again to the relaxation field for another τ/2 period, and finally (f) switch once more to the observe field and acquire/accumulate the modulus of the echo. While this is technically remarkable and merits attention, I admit that I do not feel quite comfortable with the poster's title since, as always, the intensity of a single spin-echo is not affected just by T2, but also by diffusion and flow across field gradients, J-coupling, and chemical exchange.
Stelar, still the only producer of commercial FFC-NMR hardware, was the only exhibitor present at the meeting. They were showing the new SMARtracer, a compact (bench-top) field-cycling NMR relaxometer suitable for both routine and research applications.
|
May 27, 2007
P.C.Lauterbur
P.Mansfield
R.V.Damadian
R.Ernst
| The departure of Paul C. Lauterbur
Prof. Paul C.Lauterbur, one of the inventors of Magnetic Resonance Imaging (MRI) for which he was awarded the 2003 Nobel prize in Medicine, has died on March 27 this year shortly before reaching his 78th birthday (May 6).
In my own ageing memory, the invention of MRI in early 70's has not been such a dramatic moment in history as people tend to think today. Actually, it came up belatedly since for many physicists conferring spatial resolution to NMR experiments had been even then a rather obvious possibility, often mentioned during informal talks and sometimes even in research papers. Two approaches used to be cited in that connection: a small (focused) area of homogeneous magnetic field typical of any magnet, and the use of magnetic field gradients. What did not click were two things: First, the idea that such localization techniques could be handled in a dynamic way and refined to the point of obtaining a complete image of the internals of an object rather then just discriminating between two of its areas. Second, the very idea of magnets large enough for a human body seemed something akin to a drunkard's delirium. I remember that, when I have first heard about NMR investigations of human bodies (around 1978), I was awe-struck by the magnet while the principle of obtaining the images barely aroused my curiosity. Even after medical applications started appearing, it took a few more years before I have fully appreciated the importance of MR for medicine and corrected my opinion that "there would never be more than a dozen machines in the whole world".
Paul C.Lauterbur had neatly removed the first obstacle by devising a way of arranging the data acquisition & handling so as to obtain a detailed map of the investigated object. The fact that he was a chemist may explain why he was sensitive to the possibility of investigating chemical inhomogeneities withing microscopic (hic) samples and why, apparently, even he needed some time to make the transition from microscopic to macroscopic samples and to appreciate the consequences of his own work for medicine. He was also an accomplished NMR spectroscopist - his pioneering work on 29Si and 13C NMR spectroscopy, done in the decade preceding (!) the advent of Fourier transform techniques, was most fascinating and should not be forgotten because of his later achievements.
He dubbed his 1973 image-formation technique zeugmatography (from tomography, or imaging of sliced objects, and the Greek zeugma, meaning yoke, normally used as a linguistic term but here intended jokingly to refer to the yoke of a magnet); today we would classify it as a radial, multi-scan k-space sampling technique. It used a field gradient which was kept constant during a scan but rotated to a different direction between one scan and another. The spectra of the acquired signals were thus essentially projections of proton density onto the gradient direction and, once a set of such projections was collected, the image was recovered by a projection-reconstruction algorithm borrowed from X-ray tomography. The term zeugmatography survived for about two decades in contexts such as rotating frame zeugmatography but eventually faded out in favor of the acronym MRI.
There are numerous possible alternatives to the zeugmatography scheme, many of which were independently explored in the UK by Peter Mansfield who was awarded the Nobel prize jointly with Paul Lauterbur. The subsequent febrile accumulation of various techniques by an ever growing number of researchers (including Richard Ernst, another Nobel prize winner) culminated by the introduction in 1983 of the k-space formalism (S.Ljunggren and D.B.Tweig) which allowed their rational classification and optimization and made manifest the infinity of ways how the goal of forming an image can be achieved. Today, the early zeugmatography of Paul Lauterbur looks amusingly simple and inefficient, but back in 1973 it was certainly quite revolutionary and unique.
There was another prominent man associated with the very earliest MRI, namely Raymond V.Damadian. Technically, his approach was far less sophisticated than those of Lauterbur and Mansfield. He started with a variety of the homogeneous-point spatial localization, which he called "fonar", in analogy to "sonar", the "f" standing presumably for (radio-)frequency. As I see it, his contribution was more "political" than "scientific" since he labored against the second of the two obstacles mentioned above by a relentless promotion of magnetic resonance in medicine, removing the acceptance gap between physicians on one side and natural scientists and technologists on the other. This was no less important at that time than hard science. Moreover, it is a fact that Damadian formed the very first commercial MRI venture (still in business) and registered the very first MRI patent. Many tend to agree that he should be honored just as P.C.Lauterbur and P.Mansfield, though the way of conferring the honor need not be the same (which, indeed, has already happened). I mention this because of the most unfortunate and lengthy feud between Damadian and Lauterbur which has seriously damaged both of them. For one thing, it has certainly delayed the Nobel prize committee's decision regarding MRI, formalized only 30 years after the clue discoveries. The committee must have been wary of a repetition of the 1912 blunder when the inventors Thomas Alva Edison and Nicola Tesla were nominated for the Nobel prize for Physics but, due to a deep personal feud, both have refused to accept it jointly with the other.
Unlike Sir Peter Mansfield, Paul Lauterbur did not stay with MRI forever. In later years he became interested in hypotheses concerning the origin of life, contemplating the probability of its spontaneous genesis in complex chemical systems.
Selected References:
- Holzman G.R., Lauterbur P.C., Anderson J.H., Koth W., Nuclear Magnetic Resonance Field Shifts of Si29 in Various Materials, J.Chem.Phys. 25, 172-173 (1956).
- N.Muller, Lauterbur P.C., Goldenson J., Nuclear Magnetic Resonance Spectra of Phosphorus Compounds, J.Am.Chem.Soc. 78, 3557-3561 (1956).
- Lauterbur P.C., C13 Nuclear Magnetic Resonance Spectra, J.Chem.Phys. 26, 217-218 (1957).
- Damadian R., Tumor Detection by Nuclear Magnetic Resonance,
Science 171, 1151-1153 (1971).
- Lauterbur P.C., Image formation by induced local interactions: examples employing nuclear magnetic resonance, Nature 242, 190 (1973).
- Lauterbur P.C., Kramer D.M., House W.V.Jr., Chen C-N., Zeugmatographic High Resolution Nuclear Magnetic Resonance Spectroscopy. Images of Chemical Inhomogeneity within Microscopic Objects, J.Am.Chem.Soc. 97, 6866-6868 (1975).
- Kumar A., Welti D., Ernst R.R., Imaging of macroscopic objects by NMR Fourier zeugmatography,
Naturwissenshaften 62, 34 (1975).
- Kumar A., Welti D., Ernst R.R., NMR Fourier Zaugmatography,
J.Magn.Reson. 18, 69-83 (1975).
- Mansfield P., Maudsley A.A., Baines T., Fast scan proton density imaging by NMR, J.Phys.E:Scient.Instrum. 9, 271 (1976).
- Twieg D.B., The k-trajectory formulation of the NMR imaging process with applications in analysis and synthesis of imaging methods, Med.Phys. 10, 610-621 (1983).
- Ljunggren S., A Simple Graphical Representation of Fourier-Based Imaging Methods, J.Magn.Reson. 54, 338-343 (1983).
- Click here for a complete list of MRI books.
|
May 21, 2007
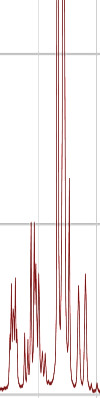
| Advances in computer-aided evaluation of high-resolution NMR spectra
This is a topic I had been [again] intensely working on during the last year and a half. I say 'again' since it used to be the object of my attention in my early scientific carrier (simulation of NMR spectra and annexed topics in Prague in the 60's) as well as - in an on/off manner later on (introduction of the automatic analysis with Peter Diehl in 1974 and the Bruker PANIC program written jointly with Jürgen Vogt in late 70's). In total, I have written simulation & fitting programs for HR-NMR spectra over and over again, totalling three different assembly languages (Zuse, Minsk, Aspect) and two high-level ones (Algol, Fortran).
Presently, in the framework of my collaboration with Mestrelab (particularly with Carlos Cobas and Nikolay Larin) I have completed the collection with a C++ version and we are presently moving fast into the vast and exciting area which lies beyond 'mere' fitting of experimental NMR spectra and structural-formula verification.
The goal of the effort is nothing less than replacing the spectra-interpretation expert with a software wizard. Which, of course, is a joke - when new tools become available, experts have a remarkable capability to re-emerge at a higher level. We do feel, however, that much more can be done in this area than what we have been so far used to. If you are interested, come to hear the invited talk I will give at the SMASH 2007 meeting (Chamonix, France, September 16-19).It is signed jointly by me and Carlos Cobas and its abstract is available both on this site and in the conference program. It can give you a more detailed idea about what we are after.
In the near future, I plan on addressing the topics of HR-NMR spectra simulation, fitting, verification, and interpretation quite frequently on this blog. Though some of the newest concepts are momentarily confidential, there is a lot of stuff which is less than original, but which has been half forgotten by many young NMR spectroscopists. I will try and tackle some of such topics in a series of brief notes and blog entries.
|
May 19, 2007
| What is the chemical composition of the ESR pitch standard
The 'strong pitch' and 'weak pitch' samples, together with the DPPH (2,2-Diphenyl-1-picrylhydrazyl) g-factor reference, have been used since decades as ESR standards. Recently I got particularly interested in the strong pitch since it gives extremely strong signal and I know from practice that it is perfectly stable (the absolute intensity of its ESR signal stays constant for at least 25 years). To my utter puzzlement, though, I can not find its composition anywhere, be it literature or internet. The closest I have ever got to its description is the following sentence in the old book by Charles P.Poole Jr., Electron Spin Resonance: A Comprehensive Treatise on Experimental Techniques, Dover Publications, 2nd Edition 1997, ISBN 0-486-69444-5:
The Varian standard is 3.3 10^(-4) % pitch on KCl. It contains 10(^13) spins per cm of length and has g=2.0028, ΔHpp = 1.7 Gauss and A (y'm ΔHpp^2) = 5.46.
Since Varian is since decades out of ESR, does anybody still know what this mysterious pitch is and how can one get hold of it? Even if it should be some natural pitch, it is clearly reproducible enough to be used as a standard. I would be most most grateful for this apparently lost piece of knowledge and willingly share it with everybody.
|
May 08, 2007
| A crop of Electron Spin Resonance (ESR) books
There are two important ESR (or EPR) books which became available in 2007 and one more is scheduled for July but can be already ordered. Three dedicated ESR monographs coming out in a few months represent quite a crop! These are the titles and their Amazon links:
Interested in more? Consult the complete list of ESR books on this site.
|
May 07, 2007
| Visit to Metrolab and magnetic field measurements
This is an overdue report on a pleasant visit to Metrolab, a Swiss company in Geneve, I have made at the end of March. I was invited by - and had a most interesting discussion with - Philip Keller and Pascal Sommer, two of the Partners of this small Company. The reason was that, though they don't develop NMR applications in the sense the readers of this blog usually intend them, they use NMR and ESR techniques to measure, plot and/or actively stabilize magnetic fields. They also produce rare earth permanent magnets which should interest many people in the NMR community, especially those developing NMR applications based on simple and/or portable MR relaxometers. Their magnets were designed for calibration purposes but that might change since they are extremely lightweight, have NMR-grade homogeneity, decent gap & access, and the Company is open to suggestions.
As I said, I have enjoyed the visit a lot, mostly because these guys are technically minded, young and dynamic. Their personal background is particle physics where magnets abound (it is no accident that they are close to CERN), but their market spreads also into MRI and even into the more traditional NMR. Their instruments provide field-mapping capabilities for magnet manufacturers and MR equipment installation technicians. In my opinion, instruments of this kind will be in the future much more ubiquitous around MR installations, both big and small, than they are today.
It was fun to see their tiny NMR front-ends with integrated preamplifiers and to find out that they "still" use CW techniques with miniature crossed-coils probes. I have also liked their unique NMR/EPR field cameras which mount an amazing array of miniature probes to precisely map extensive magnetic field regions such as, for example, the whole field-of-view (FOV) of a whole-body MRI magnet. At the same time, I could not help to feel that much more could be done if we brought this type of Companies closer to the heart of what, so to speak, is now boiling in MR kettles.
Finally, a figure on the Metrolab web page brought my attention to something else - an excellent set of slides on magnetic field measurements prepared by Luca Bottura (CERN) for a 2002 physics meeting. If you want to bone up on this topic, Luca's broad perspective and competence make his site a good starting point.
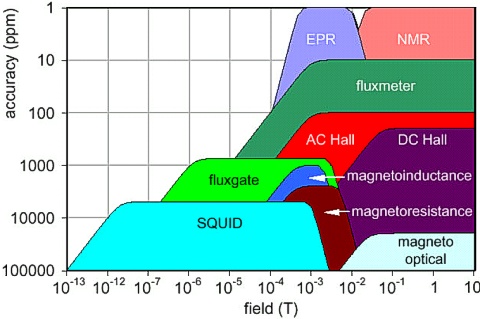
The Figure reproduced above shows the field-versus-precision diagram of various magnetic field measurement techniques. It can be extended up in a trivial way to cover all currently used NMR fields (max 35 Tesla). The EPR area could be also extended quite a bit, especially when combined with optical detection (though, commercially, that is still a fantasy).
|
April 22, 2007
| Impressions from 22nd NMR Valtice
I have just returned from the enjoyable annual meeting of the Central European NMR Discussion Groups (22nd NMR Valtice). I have missed it last year, but reported on the 20th edition two years ago. As always, the meeting was held at the Lichtenstein castle of Valtice, a tiny town in southern Moravia (Czech Republic).
This year there were 75 participants from ten Countries. Most of the contributions centered on NMR spectroscopy applications to structural chemistry. Since, given their number, I can not possibly comment on all of those (please, see the conference abstracts), I will mention only the few exceptions.
One was a talk by Norbert Müller titled Recent Progress in Spin Noise Spectroscopy. This is a novel NMR method/phenomenon based on the analysis of the thermal electromagnetic noise generated in a sample, with no excitation pulses at all - and the very fact that it works is truly amazing. Norbert did not manage to supply an abstract of his talk (deadlines), but you can download his and Alexej Jerschow's most recent article Nuclear spin noise imaging, PNAS, 103, pp.6790-6792, 2006, and follow the references therein.
As a programmer & physicist, I have enjoyed a talk by Zdenek Tosner about the Design of New NMR Experiments by Means of Optimal Control Theory, aimed at maximizing the sensitivity of complex NMR sequences. I was intrigued by some of the solutions - at first glance, the optimal pulse phase modulation plots look like random functions of time. It is an interesting topic which I would love to study in more detail.
Milan Mazur had a technical presentation on the problems of quantitative ESR spectrometry. This is an old and important topic which, alas, has no neat solution. Milan and his coworkers examined the entity of the absolute intensity errors one can commit when mis-positioning the sample with respect to the center of the resonant cavity.
Last (and least) there was my own musing about the Perspectives of Passive and Active Magnetic Resonance in Astronomy, a topic which I fancy. On this occasion I have talked mostly about the gaps in our understanding of the MR phenomenon (along the lines discussed by D.I.Hoult and B.Bhakar in Concepts of Magnetic Resonance, 9, p.277, 1997). Some of the quantum aspects of magnetic resonance which are yet to be verified have an immediate impact on how active MRA (Magnetic Resonance Astronomy) should be carried out and what new phenomena might be encountered (I will return to this theme in a full article).
The meeting had a rich social agenda which culminated with an excursion to Lednice, an area including a Lichtenstein mansion with a large greenhouse, a park and even a minaret (the whole complex is on the UNESCO World Heritage list). Another memorable moment was an evening at the Czech National Wine Center, located in the wine cellars of the Valtice castle, which currently offers samples of 83 carefully selected wines produced in the southern Moravia wine-growing region.
This meeting merits attention for its high quality, and also because there is not much else going on, in NMR terms, in the European countries which once belonged to the East Block. Thanks to the organizers from Masaryk University and Pliva - Lachema a.s. (both in Brno), and from the Czech Society of Industrial Chemistry.
|
April 20, 2007
| MR safety: Need for caution cannot be overstated
A reader has sent me an e-mail which definitely merits a wider attention:
I recently learned of a serious personal injury that resulted from the attempt to use a ferro-magnetic screw driver when repairing an NMR at [ withheld ]. Apparently the injury resulted in the course of efforts to recover the screw driver after it was captured in the NMR. The incident involved penetrating frontal lobe injury to the engineer.
The title of this entry matches the subject of the signed e-mail. I have withheld the sender's name and the name of the Institution where the accident happened (it was one of the most prestigious high-tech establishments in California). The message illustrates three facts:
1. Accidents do happen quite often and nobody is immune.
2. They are invariably due to human errors and, most often, outright foolishness.
3. They happen even to experienced technicians who should know better.
One of the problems of controlling ferromagnetic objects located within the magnetic fields of MR magnets (be they part of superconducting spectrometers or of MRI scanners) is that, in many ways, they do not behave as our instincts tell us they should. This is due primarily to the fact that the force exerted on them (and thus also the counter-force required to keep them in place) is proportional to the field gradient rather than to the field strength.
Consequently, the force is null in those areas where the field is homogeneous (typically inside the field-of-view), regardless of the field magnitude. On the other hand it can be totally overpowering (even for objects as small as a screwdriver or scissors) in an area close to the ends of the magnet where the field is rapidly decreasing and its gradient is large.
For the same reason, the maximum force can be similar for a small 1.5 T magnet as for a large 4 T magnet of a similar make. This is because the field of the larger magnet, though stronger, tapers off over a longer distance, a fact which makes the gradients comparable. However, there is a large difference between the new, actively shielded magnets and the older, unshielded ones. The shielded magnets feature considerably reduced stray fields which implies that, close to the magnet, there is a transition region where the field rises toward its central value much faster than in an unshielded magnet, causing the force exerted therein on a magnetic object to be many times larger (though limited to a smaller volume).
Another reason why we are so often taken by surprise by these forces is their unusual dependence on spacial coordinates. The field of a magnet (at least an unshielded one) is similar to that of a magnetic dipole and thus, moderately far from the magnet, tapers off with the third power of distance from the magnet's center. For the gradient, this means that it falls with the fourth power. Consequently, when one approaches a magnet while holding a [saturated] ferromagnetic object, the exerted force is first comfortingly small and then, quite unexpectedly, shoots up as though the magnet had abruptly decided to snatch the object away. Since our minds are accustomed to linear dependencies, not to fourth powers, we either let go of the thing (since it also tends to twist in the field direction, it feels as though it had suddenly become alive) or else we over-react violently in yanking it back. Either way, we are out of control.
There is much more to these things one could write about. Like elongated objects getting oriented and flying into the magnet like javelins. Or non-magnetic metallic objects being strongly hindered by induced eddy currents. But the main point is just one: When handling magnetic objects inside magnetic fields, nobody can trust one's instincts - and when things start happening, even the expert has no time to take advantage of his/her rational training!
I want to conclude this entry by reporting another accident I have been told about (fortunately, nobody got hurt in this case). It involved a gas cylinder which ended up in the bore of an MRI magnet. Trying to get it out, the 'technicians' enlisted the help of a small motor fork-lift. They strapped the cylinder and tried to tie it to the fork lift by a rope. Unfortunately, the rope was too short, so the fork-lift operator had to 'cautiously' back towards the magnet. They figured that if they backed just an inch at a time and kept checking whether everything was ok, they would be safe. Until, of course, after one more backward jerk the fork-lift got suddenly air-born and smashed into the entry port of the magnet (fortunately, the driver fell off). It then took a much larger fork-lift, and a bunch of very long tow-ropes, to detach all the stuff from the magnet (which was another very foolish thing to do, but they were lucky and got off with it). Suprisingly, the magnet, though badly scratched, did not quench and remained functional.
As a final memento, have a look at this photo gallery of MRI mishaps.
|
April 15, 2007
| eDISPA: New approach to automatic phasing of HR-NMR spectra
Together with Carlos Cobas of Mestrelab Research, I have developed a novel, automated method of phasing HR-NMR spectra (or frequency-domain NMR data, if you wish). The method, rooted in an extension of the old DISPA plots (Dispersion versus Absorption), is described in a poster to be presented at the forthcoming 48th ENC Conference (Daytona Beach, Florida, April 22-27, 2007). It is now being implemented in the new MestreNova software package. Since it is a parametrized algorithm, it may take a period of practical field-testing before the parameter values for various kinds of experimental data get fine tuned, but the results obtained so far look extremely promising.
Those interested in this matter are invited to download the poster (PDF, 260 KB) in advance and/or track Carlos at the meeting to discuss it with him (unfortunately, I can't attend). Also, the eDISPA poster page on this site carries a complete list of titled references concerning the DISPA plots and their applications.
|
April 07, 2007
| Starting an RSS service
For a long time, readers kept asking me to implement "an RSS button" on this blog and, for just as long time, I was avoiding the topic because I thought that the Real Simple Syndication concept was sure beyond my comprehension. Finally, with the help of a wise friend, I have decided to look at it and, in terms of simplicity, it turned out to be up to what it says (at least in theory, now let's see it in practice). So, those of you who know what to do with it, here comes The BUTTON !
I am starting the service with 15 recent items, almost all of which are picked from this NMR blog and from Stans Library, the two sources which will remain its core. However, I will occasionally add also external items which attract my attention. Likewise, if you would like me to syndicate an MR-related announcement, please, let me know. Particularly welcome are links to on-line educational materials, posters and articles, as well as announcements of new MR Groups, Societies, Events, Companies and innovative products.
|
April 02, 2007
| NMR Encyclopedia mystery solved
Regarding the previous entry, there is no better way to solve a mystery than getting hold of the chief culprit. I have contacted the Editors and received the following answer from Robin Harris:
Dear Stan,
Thanks for your message. I am amused that you have picked up on what is clearly a rumour founded on substance but rather misleading. However, I can readily clarify the situation for you so as to allow you to update your blog - which, in view of the "mystery" and the heavy visitation of the blog, would be highly useful.
The fact is that a new PRINTED edition of the encyclopedia is not in question until 2010 at the earliest. However, there is very considerable activity on the Encyclopedia at present, in three related ways:
1. All the current articles are being put on the web, with the topics searchable. In fact this process is now (just) complete, and I presume Wiley will be proudly announcing this. One effect is to cope with your comment about the cost of the current 9-volume set, since the individual articles will be available on a pay-per-article basis.
2. Secondly, we are in the process of planning to bring the Encyclopedia up to date by (a) updating individual articles where this is needed (some articles are clearly more timeless than others), and (b) adding entirely new articles to cater for recent developments. All changed and new articles will be electronic, of course, with "replaced" articles still being available in the background.
3. We are planning to gather together Encyclopedia articles with a common theme, to be published in print versions as "Handbooks". In fact the titles of the first 4 handbooks have been decided. Priority choice of new & updated articles under point 2 above will be directed towards the designated handbooks - and of course process 2 therefore has precedence. We are hoping the first handbooks will appear in 2008. This initiative will again help with the financial issues you have quite correctly raised.
We have a partially-new set of editors for the Encyclopedia. I continue as an Editor-in-chief, and am joined in that role by Rod Wasylishen. Dave Grant becomes Chairman of the International Advisory Board.
Regards, Robin.
Thanks for the detailed clarification, Robin. The Encyclopedia is an important Opus, despite the fact that I will probably continue to see most of it only through shopping windows (unless somebody buys me a couple of the articles for Christmas).
|
March 30, 2007
| NMR Books and Pyramids
Somehow, books keep catching my eyes in this period. There has been an announcement on InterScience of a new edition of the Encyclopedia of Magnetic Resonance, edited by D.M.Grant and R.K.Harris and published by J.Wiley. The announcement was picked up also by the NMR Knowledge Base, but it is not at all clear from the announcement whether this is a re-edition of the 9-volume set or whether new material - such as a new volume - has been added. Paradoxically, searching for the title on the J.Wiley site does not yield anything more recent than the 9th volume of 2002 ($945) and the 9-volume set of 2003 ($7375). Even more amazingly, searching Google for the presumed ISBN (9780470034590 or 0470034590) yields nothing at all !!! Which makes the new edition a bit of a mystery.
While this is probably just a consequence of a hasty announcement, I was wondering about the usefulness of books costing a thousand dollars apiece. Agreed that it is an Encyclopedia, but who can afford it !?! Not a student, for sure, nor a honest, free man living in a 'developed' country like me. Which makes it akin to a monument to the old boy's glories and a repository for the moldy mummy of past achievements. Very much like the pyramids - except that the hope of a final resurrection is even fainter in this case.
There is no doubt that the editors' work on this Pyramid was as extenuating as that of the Egyptian slaves, and that they have done an equally superb job. It would have been fantastic if the work had been subsidized by one of the many Agencies for the Advancement of Mankind and sold for $1 per set. As it is, however, we plain mortals will have to do with admiring it from the outside on guided tours.
The complaint actually applies also to other books and, especially, Scientific Journals. There are many Institutional initiatives aimed at the promotion and advancement of Science. Some of these are very costly and there is little doubt that some are even useful. But nothing could be more efficient in promoting Science than making sure that every individual anywhere on this planet can afford access to the entirety of scientific literature. With present technical means, the costs would be but a tiny fraction of those of even the smallest of wars!
|
March 20, 2007
| Fresh off the presses: Molecular and Cellular MR Imaging
Much as I dislike promoting a single book rather than all of them, this one really captured my attention. Edited by Michel M.J.J.Modo (Uni London) and Jeff W.M.Bulte (Johns Hopkins) and published by CRC Press, it provides the first overview of a new MRI & MRS branch, the cellular and molecular imaging, which goes a long, long way beyond routine picture-shooting of a person's insides. This excerpt from the book's abstract explains why it is so exciting:
"The ability of molecular and cellular imaging to track the survival, migration, and differentiation of cells in vivo as well as monitor particular gene expression in living subjects is rapidly moving from the research laboratory into daily clinical settings. The interdisciplinary nature of the field mandates a constant dialogue among molecular & cellular biology, chemistry, physics, image analysis, and drug discovery to develop and translate promising approaches into reliable scientific applications and viable clinical diagnostic tools."
The book, slated for distribution by the end of this week, is not particularly cheap but it is worth the money. You can already order it from Amazon.
|
March 6, 2007
| X-domain NMR
I suppose that you have noticed the recent drift towards the terms time-domain NMR and frequency-domain NMR in place of the oldish high resolution NMR (HR-NMR) and low-resolution NMR (LR-NMR). There appears to be a determined drive towards these terms, as though somebody influential enough were pushing them (which, probably, is the case).
For me, this is fine since I was never comfortable with the LR-NMR denomination. Having spent much of my life in that area, I always felt that 'low-resolution' sounded unfair and derogatory and I often felt compelled to explain that it referred to the nature of the investigated system rather than to any lack of money to buy a decent magnet (even though that often was a factor, too) or a computer to do the FFT.
In late 70's, when I worked at Bruker, they also felt uncomfortable about the terminology. At that time there still existed two distinct departments. Before the advent of FT-NMR (in 1968-1970), one department (headed by Bertold Knüttel) was referred-to as pulsed NMR while the other (headed by Tony Keller) was labelled continuous-wave NMR, so the distinction centered on excitation-detection methods. After the FT, however, NMR spectroscopy became also pulsed and the department names had to change. The preferred terms became the well-established high-resolution for Mr.Keller's department, while Mr.Knüttel's stuff started to be labelled as solid-state NMR (a distinction based on the prevalent nature of the samples) or as high-power NMR (the required transmitter power was only 100 W for high resolution but 1 kW for solids).
I should also mention wide-line NMR which denoted continuous wave NMR instruments for solids. I used one of those, produced by Varian, back in 1973 at the Analytical Chemistry Department of the University of Illinois in Champaign-Urbana, to measure the NMR spectra of poly-crystalline powders of some acetates. They spread over about 20 Gauss (oops! 2 mT) and they were absolutely beautiful. I have never seen such a high-quality, real solid-state NMR spectra ever since. Which, I guess, is why everybody stopped producing those instruments and now you can just dream about them while fighting "terrible" dead-time problems. Ha,ha!
In the 80's, history started playing more terminology tricks. With the advent of magic angle spinning (MAS) and cross-polarization (CP), the meaning of the term solid state NMR started narrowing down to the CP-MAS-of-low-abundance-nuclides niche. And I underline niche because that's what it is, despite the fact that most chemists consider it the better half of the Universe. Misguided as they are (:-), they don't wont to understand that real solid state NMR is much more! Maybe we should start distinguishing systematically between solid-state NMR and NMR of solids.
So, returning to my little story, Bruker had to drop the solid state NMR label for Mr. Knüttel's department. Moreover, the high-resolution solid state NMR also started using quite high transmitter powers, so they had to drop the power-level distinction as well. Eventually, they dropped the whole two-departments concept and re-organized the Company!
Which is quite characteristic of NMR: it keeps sprouting new branches all the time but it also makes sure to keep them united and inter-twinned. One can never make a distinction and hope that it will last for more than a decade. I confidently assume that this is exactly what will soon happen also to the frequency-domain versus time-domain alternative.
For one thing, the way we do NMR today, we always start with time domain data anyway. Since the computer is also always present, whether we apply the FFT and move to the frequency domain is just a matter of o few kBytes of code and the willingness to hit a key. In addition, we have also other "dimensions" to consider. What about space-domain for the spatially resolved MRI, relaxation-rate domain for relaxation-ordered techniques, and diffusion-rate domain for diffusion-ordered methods. Not only we witness all those cases coming up vehemently, we also see all possible combinations between them.
So it may well be time to start once more groping for some new terminology!
|
March 1, 2007
| An FFC-NMR relaxometry review made available online
If you are interested in variable-field NMR relaxometry, have a look at the Note added at the bottom of this page.
|
February 28, 2007
| An exciting APS meeting I have missed
I have just discovered that only five days from now, in Denver, Colorado, starts the March Meeting of the American Physical Society and that one of its Sections (V3) is entirely dedicated to Detection & Applications of NMR and MRI at Microtesla Magnetic Fields. The five invited presentations look all extremely exciting and regard topics I have either already written about or intended to do so as soon as possible.
One of the talks, Simultaneous Measurement of Magnetic Resonance and Neuronal Signals (M. Espy from the Los Alamos National Laboratory), is directly pertinent to the theme of my last entry (February 21). Actually, by combining SQUID detection of MR brain signals with that of neuronal currents, it goes a step beyond what I was worried about.
Three contributions, respectively by M. Moessle, S. Appelt (both from UC Berkeley) and M. Burghoff (from PTB-Berlin), point in the direction of a very feasible, and very usable, high-resolution NMR spectroscopy in very low magnetic fields (such as the earth field), a topic which is presently as hot as a supernova (I have seen recently some really complex earth-field NMR spectra with tens of 0.1 Hz wide lines).
The last talk, Optical methods for detection of nuclear magnetic resonance by M. Romalis from Princeton University, regards a recently discovered spin-optical phenomenon (a non-resonant rotation of the polarization plane of an electromagnetic wave by polarized nuclear spins).
I want to return to the last two topics since they bear the marks of revolutionary breakthroughs. Which is a reason why, as a physicist, I really regret that I can't be present at this meeting.
|
February 21, 2007
| Can fMRI become a new brain-snooping gadget?
It certainly looks so, considering the recent shocking article by J.T.Kaplan, J.Freedman and M.Iacoboni entitled "Us versus them: Political attitudes and party affiliation influence neural response to faces of presidential candidates" which appeared in the January 2007 issue of Neuropsychologia (Volume 45). Despite its misleadingly humorous title, the study proves that functional MRI has the amazing capability of detecting and resolving subconscious emotional reactions to external stimuli. Specifically, this is what the Abstract of the article says:
We investigated how political party affiliation and political attitudes modulate neural activity while viewing faces of presidential candidates. Ten registered Democrats and 10 registered Republicans were scanned in an event-related functional MRI paradigm while viewing pictures of the faces of George Bush, John Kerry, and Ralph Nader during the 2004 United States presidential campaign. We found that compared with viewing one's own candidate, viewing the candidate from the opposing political party produced signal changes in cognitive control circuitry in the dorsolateral prefrontal cortex and anterior cingulate, as well as in emotional regions such as the insula and anterior temporal poles. BOLD signal in these regions correlated with subjects' self-reported ratings of how they felt emotionally about the candidates. These data suggest that brain activity when viewing a politician's face is affected by the political allegiance of the viewer and that people regulate their emotional reactions to opposing candidates by activating cognitive control networks.
Reports about such technical capabilities started appearing several years ago [click here] and there were also several very recent comments (click here) on the specific study mentioned above. It appears to me, though, that these comments over-stress the bizarre traits of the matter, while missing the fact that what we are witnessing here is the birth of a high-resolution emotion-scanning technology - something that might have social implications far beyond those of the relatively primitive polygraph machine. There is also no doubt that the technology is both viable and rapidly evolving (the buzz words in this context might become SQUID detectors and low-field MRI using innovative, casket-shaped magnets).
It is not at all difficult to imagine powerful political personalities, public administrators and business managers requiring their collaborators to undergo fMRI loyalty tests. Likewise, an individual's sexual orientation, racial and religious tolerance, team spirit, patriotism and even attitudes towards abortion or adultery might soon become objectively testable.
Clearly, this opens a whole category of ethical questions and will soon lead to the need for specific legislative actions. In this context, meetings like the pioneering Round Table on Appropriate Use and Ethics of Medical Imaging (an EMRF initiative to be held on May 25-26, 2007 in Berlin) might play an important role, giving MR researchers and practitioners an opportunity to affect things before they are taken out of their hands and put into those of politicians and legislative bodies.
|
February 14, 2007
| New desktop MRI and FFC instruments
Recently, I have stumbled on the site of a new Israeli MRI Company named Aspect. The site is very recent and so far consists of a single page - I have found it only because the Company name resembles the legendary ASPECT computers of Bruker. Puzzled, I wrote to the Company management and received an interesting answer which I want to share with you, hoping that my readers and Aspect competitors will not accuse me of unfair advertisement (take it as an info rather than an endorsement):
Dear Stan,
Thank you for your e-mail. Indeed, Aspect is developing an innovative desktop size 1T-1.5T MRI system for various applications. The system can be placed in almost any environment due to its highly efficient design and the property of zero magnetic fields outside the system. We are operating through 2 companies:
Aspect Magnet Technologies - focuses on pre-clinical and clinical applications
Aspect AI - focuses on agriculture, industrial and energy applications
Both companies are in business and expect beta installations and initial sales this year.
Our website will soon be on air. Meanwhile, I summarize the major properties of our new MRI system for your website and I think it should fit also the MRI manufacturer's category.
The Aspect MRI system has clear competitive advantages over existing MRI solutions by offering the users unique performance coupled with unparalleled cost effectiveness:
- High performance medical and industrial grade solution:
1 - 1.5 T, up to 20 micron resolution depending on the application
- Has equivalent or better performance than systems with stronger magnets due to better S/N
- Desktop size apparatus and fully mobile - examines objects/animals/organs anywhere
- In-line or on the production line installation
- High scanning speed - 9 objects/sec.
- Zero external magnetic fields:
can operate in almost any conventional environment, no need for shielded room
- Convenient operation
low maintenance, room temperature operation, low power, no gradient noise
- Upgrade-able, modular solution (different field intensities, different features)
- Simple to use: no need for specially trained technicians
- Affordable:
System at a cost never before
Minor operation costs
Aspect novel technology implemented in our new MRI systems provides solutions to some of the most important parameters - performance, pricing, mobility, operation and simplicity. We believe it will bring MRI technology to many new users.
I'll be happy to keep you updated on our progress.
Sincerely, Oz Bornstein, VP Corporate Development, Aspect Magnet Technologies
-------------------
So, if I get it right, we have here a very compact MRI system commercialized by two sibling Companies in two distinct application areas.
What I find interesting is the fact that this is one of several recent initiatives based on two key features: bench operation combined with an innovative magnet. The second such case is the SMARtracer NMR relaxometer manufactured by Stelar, where the magnet is of the fast-field-cycling (FFC) type. Considering also the NMR and MRI instruments of Magritek and some of the NMR MOUSE devices, we witness the emergence of a whole category of desktop-to-mobile NMR & MRI instrumentation in which differences between individual products consist primarily in the type of magnet. I have no difficulty, in fact, to imagine a universal electronics + software package running all of them (challenge me and I might set it up!).
-------------------
A reader has complained that too much of my blog space is dedicated to technological and commercial aspects of MR. This may be true - but only because there is so much fresh activity in several non-traditional NMR and MRI areas addressing new challenges and opening novel applications. I promise to return to pure Science (whatever that means) in the next entries.
|
February 10, 2007
| Update on the NMR process-analyzers scene
In the last paragraph of the January 28 entry I wrote that "I perceive an ongoing turmoil in the area vaguely described by the term process analyzers". John C. Edwards, a manager at Process NMR Associates, has kindly taken up the challenge of updating me on the current situation. It turns out that, not being an insider and having based my comments on web sources which are often neither complete nor up-to-date, I have commited a few errors. I therefore want to share his comments and corrections with you (all I have added are the links):
Hi Stan
I noticed your piece on MR companies appearing and disappearing in your blog. I just wanted to say that there is no turmoil in the process NMR area, just an expansion of the number of companies supporting that market.
Invensys is marketing the Qualion NMR Analyzer as are a number of European and Chinese process analyzer companies. Process NMR Associates is also marketing Qualion NMR analyzers to several markets through a joint venture company called NMR Process Systems. This joint venture company comprises Process NMR Associates (bringing NMR and process analytical background) and TTC Labs (providing engineering services). FYI - Donahue Process Systems no longer exists .... it was replaced by NMR Process Systems about a year ago.
The Qualion NMR systems are fully shimmed 60 MHz high resolution FT-NMR spectrometers producing spectra that are chemometrically modeled to produce calibrations capable of predicting many chemical and physical properties used to control processes in industrial manufacturing.
On a completely different business track Process NMR Associates is marketing the Spin Track analyzers from Resonance Systems Ltd in the United States. These are time domain NMR spectrometers that are currently available for laboratory or mobile testing. They could be used for online TD-NMR applications but currently we are not pursuing that avenue...though I do know that Resonance Systems have been performing on-line analysis with other customers of theirs.
Cheers, John
|
February 2, 2007
| A new source of super-paramagnetic particles
Soluble ferro- and superpara-magnetic particles are becoming an increasingly popular tool in NMR relaxometry and in MRI. In MRI they are used mostly as contrast agents, while in NMR relaxometry they can be exploited to create strong random magnetic field gradients and thus, for example, discriminate small, highly mobile molecules from large ones in sequences based on spin-echo detection.
For this reason I have listed a new Company, MagSense Life Sciences Ltd (West Lafayette, Indiana, USA), in the NMR Consumables section of my MR Companies directory. They produce hydrophile, spherical super-paramagnetic particles of uniform size (1 μm) at a quite affordable price. Since the spherules are used principally for molecular separation in biochemistry, the MagSense site does not even mention NMR or MRI among their applications. Which does not mean that they can't be used!
|
January 28, 2007
| Companies popping-in and popping-out
There is always something new happening in the MR Companies landscape - a fact which kept my interest awake during the whole of 2006 while I kept discovering relatively new but little known Companies. At the same time, completely new Companies were being started and, in at least one case, old Companies were being dismantled.
Regarding the latter case, I am somewhat saddened by the fact that I finally had to expunge from my site any reference to the ex UK Company Resonance Instruments which has been swallowed by Oxford Instruments and, over a couple of years, apparently digested with not a bone left. Whether the act was friendly or not and, consequently, whether we should rejoice or not, is beyond my knowledge (a couple of timid queries I made to find out remained unanswered).
On the other hand, it was a great pleasure to list ABQMR Inc (Albuquerque, NM, USA) set-up by Andrew McDowell and Eiichi Fukushima, the author of Nuts and Bolts. The venture (a commercial spin-off from the non-profit New Mexico Resonance) provides technical MR expertise and services, particularly in the areas of time-domain NMR and MRI.
Another venture I have found interesting is the first-ever (as far as I can tell) commercial generator of hyper-polarized 129Xe for NMR and MRI produced by Toyoko Kagaku (Japan). With the rapidly growing number of interesting MRI and NMR applications involving hyper-polarized gases, a venture of this kind should have a bright future.
I was quite a bit intrigued by Measurand Inc (Fredericton, NB, Canada), a Company which produces data gloves and limb trackers for use in all kind of science-fiction stuff. It turns out that they found a market niche in MRI (sensing patient's movements) and engineer some of their products in MRI-friendly (or, more precisely, magnetic-field-friendly) fashion. Wow!
I perceive an ongoing turmoil in the area vaguely described by the term process analyzers which includes time and frequency-domain NMR instruments for industrial plants (mostly petroleum and food industries). It involves Process NMR Associates (PNA, Danbury, CT, USA), ex Foxboro NMR, and the new Resonance Systems (Yoshkar-Ola, Russia). Foxboro NMR used to make part of the Foxboro - Invensys group and collaborate with PNA (see the July 15, 2005 entry), but all these associations were discontinued late in 2005 (see the October 26, 2005 entry). Foxboro NMR is now continuing on its own under the name Qualion NMR Analyzers (Haifa, Israel), concentrating on FT-NMR industrial plant analyzers. On the other side, PNA has recently announced a close collaboration with the newly formed Resonance Systems. There is also a close connection between PNA, Donahue Process Systems (a small Company with NMR know-how in Woodlands, TX, USA) and TTC Labs (a larger enterprise with process-control know-how in Fond du Lac, WI, USA). In other words, this area continues to be a complex and ever-shifting kaleidoscope.
|
January 1, 2007
| Let us build a Large Magnetic Room
At various occasions during the last year I have perceived a growing need to apply a magnetic resonance methodology (spectroscopy, imaging, relaxometry or diffusometry) to objects which are too large or too unwieldy to fit into a normal instrument. Here are a few examples:
- ** Large animals such as horses, cattle, elephants, dolphins, whales, etc.
- Veterinary MRI is now on an upswing and MRS will no doubt soon follow. Though nobody expects to routinely scan all ill animals, there are many special cases and situations when a scan is extremely desirable, no matter how costly it might be. Which is easy when the animal is small, but becomes quite complicated when its size is larger than that of a human. Not to speak about the fact that many large animals would be best scanned while standing.
- ** Large plants such as trees
- At the Wageningen Large-scale NMR Facility, some time ago, they have grown a little tree inside a supercon magnet so that its growth could be monitored by NMR. This kind of interest stems, among other things, from the fact that plants - trees in particular - are fascinating hydraulic systems whose lymphatic flows follow interesting 3D patterns. Today, unfortunately, any living plant whose dimensions exceed a few decimeters - and any segment of a plant larger than a human torso - represent a big technical problem, leaving out many interesting objects.
- ** Rocks
- NMR relaxometry of porous media is now an established technique with rapidly increasing importance in fields like geology, petrology, sedimentology, archeology, etc. In this context, one of the most ubiquitous problems is that a sample of a few centimeters across (which is today considered quite large) is hardly statistically representative of a rock formation (especially when it comes to dynamic phenomena such as wetting and permeation). There is no doubt that a slab of a few meters across would be infinitely more satisfactory for the purpose.
- ** Micro-crack systems in large objects
- Detection and mapping of crack systems is an area where NMR methods might soon make new inroads. One promising approach is saturating the object with 129Xe (possibly pre-polarized) and applying a combination of NMR methodologies. This is fine when you have a modest-size bone or a small meteorite. Problems arise when the sample is a larger-than-life statue or a 2x2x4 m slab of high-quality marble still to be converted into an artist's masterpiece.
- ** Archeologic objects, including unwieldy mummies
- MRI of mummies is an established practice. It is also quite straightforward when the mummy has been nicely composed by Egyption or Aztec priests. It fails, however, in cases such as the Oetzli man who got mummified with his arm stretched at an uncomfortable angle and can not be fitted into a normal scanner. Nor can one scan a mammoth frozen in a block of Siberian permafrost.
When the 'sample' is too large to fit into a standard instrument's magnet, we nowadays try and reverse the situation by combining an inside-out magnet with a specially built MR instrument and placing them close to or inside the investigated object. The first case is covered by all the novel ex-situ methods used to study surface layers of large objects, while the second one is presently exemplified by the MR well-logging techniques used to explore rock formations by sending NMR instruments down through drilled holes. With advancing miniaturization, however, I confidently expect to see soon complete MR systems fitted on a microchip and combined with rare-earth permanent magnets the size of a thimble, thus opening the road to non-invasive MR investigations of various internal passages in the bodies of large animals, including humans.
Despite these exciting new vistas, however, it is clear that the combination of a large object with a small magnet has unsurmountable, intrinsic drawbacks. We can build transmit and receive coils of virtually any size but when the magnet is small then, unavoidably, so is the field-of-view (FOV). It follows that a really large magnet - one with a useful FOV the size of an operating room - would open a number of exciting research avenues and applications. I am sure that you can all come up with examples of its potential uses - and also that the range of such applications exceeds MR and covers many other fields.
Of course, we already have one really large and homogeneous permanent magnet, which is the planet Earth. The problem is that the Earth magnetic field of about 50 μT is too tiny for many MR investigations. Even though Earth-field MR is presently advancing at a nearly explosive rate thanks to the advent of ultra-sensitive SQUID detectors, there is little doubt that a higher field, such as 0.5 T, would be much more 'comfortable'.
So these are my proposals:
- (1) Setting up a database of potential applications.
- Let us collect a database of ideas regarding the potential applications for a Large Magnetic Room. If you have an application idea, no matter how far-fetched and no matter in which field, let me know. I will collect them, sort and classify them and, once the collection is presentable, publish it on a yet-to-be established forum.
- (2) Launching a European LMR project.
- This whole idea has the characteristics of an interdisciplinary project suitable for an [at least] European-wide cooperation. I do not expect that there will ever be a Large Magnetic Room in every County/District in the world. Though I confess that back in 1980, in a conversation with Hanspeter Kellerhals (then the CEO of Bruker Spectrospin at Fällanden, Zürich), I have 'predicted' that the total of whole-body magnets ever to be installed would be five - one per continent! LOL! In any case, we must start somewhere and it would be nice to see the first LMR to be set up in Europe. So, if you would like to adhere, let me know. Once we become a dozen of Partners from various Countries, there is nothing to stop us - not even the Brussels bureaucrats.
Final note: If, after this proposal, I become an EU living joke, so be it. Now and then somebody must come out of the closet and make a proposal which might jolt a few youngsters to life. Also, I do not make this proposal to create a niche for myself. Should somebody more qualified and younger than me wish to manage this, please, step out.
|
Archive
| For a complete list of all entries since 2005, see the running INDEX
|
|
Visitor #
ADVERTISE with us
NMR, MRI, ESR, NQR
Companies
Societies
Centres & Groups
Journals & Blogs
References
Free Texts
History
Links
BOOKS Lists
MATH | SOFTWARE
PHYSICS | CHEMISTRY
ELECTRONICS | DSP
WWW | Patents & IP
SPECTROSCOPY
MRI | NMR | ESR
Instruments
ARTICLES
MR Antenna Theorem
One-Page MR Primer
S/N Perspectives
K-space and MRI
OTHER
SI Units &
Dimensions
Physics constants
Science Links
Support this site! SHOP from here:
COMPUTERS:
Deals
Bestsellers
Accessories
Calculators
This page is SPONSORED by:
Random offers:
|